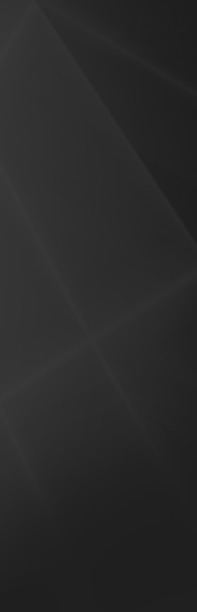
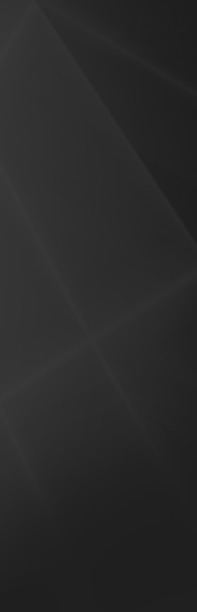
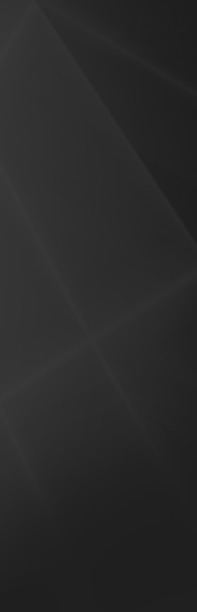
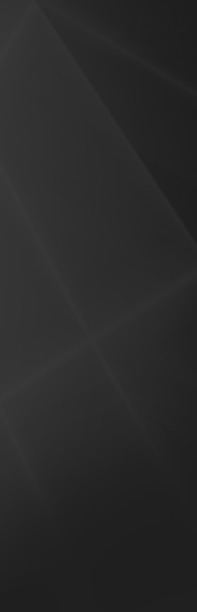
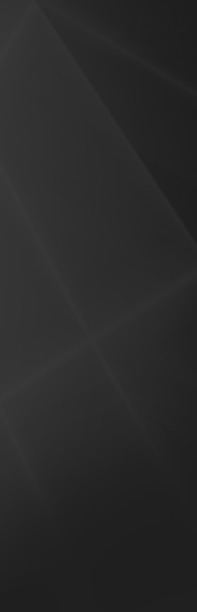
Making More Stuff
Host David Pogue hits the road to explore the frontiers of invention and innovation. Airing October 16, 2013 at 9 pm on PBS Aired October 16, 2013 on PBS
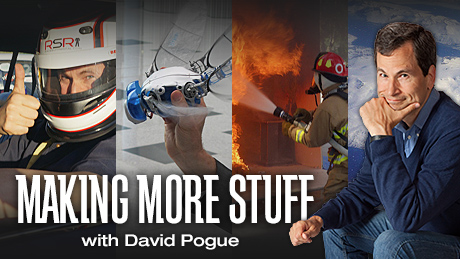
Program Description
Broadcast Credits
IMAGE:
- Image credit: (David Pogue composite images)
- Courtesy: David Pogue/Flickr
Preview | 01:12
Full Program
Full program available for streaming through
Watch Online
Full program available
Soon
Making Stuff Faster
Host David Pogue tries to find out if there are physical limits to how fast we can go. Airing October 16, 2013 at 9 pm on PBS Aired October 16, 2013 on PBS
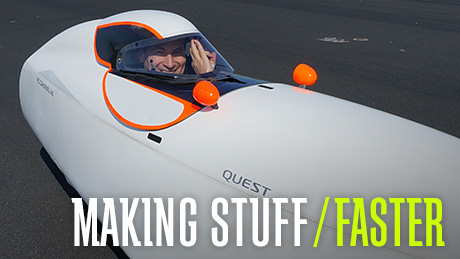
Program Description
Transcript
Making Stuff Faster
PBS Airdate: October 16, 2013
DAVID POGUE: We are living in the age of fast. Everywhere you look, scientists are discovering new ways to make stuff faster.
This is nothing! Gun it! Come on!
From ultra-fast electric cars…
It was like…
…to building machines that use human energy more efficiently than ever.
What is that?
Faster is not just about speed for speed's sake, it's also about how efficiently you can get things done, because time is money, big money.
Milliseconds are translating into millions of dollars a year?
HAROLD: It sure is.
DAVID POGUE: From discovering the quickest way to board a plane …
Man, there's got to be a faster way to load an airplane!
JASON STEFFEN (Fermi National Accelerator Laboratory): You know, there is a faster way to load an airplane.
DAVID POGUE: …to ultrafast internet connections…
CARLOS CASAS (Google Fiber Inc.): Speed tends to drive innovation.
DAVID POGUE: …going faster is transforming our lives.
Whether it's running a race, a stock trade, or exploring the universe, the history of human achievement is written by those who get there first.
NEIL ARMSTRONG (NASA, 1969 FILE FOOTAGE): That's one small step for man…
DAVID POGUE: I'm David Pogue. Join me on a quest to make stuff faster!
I'm a rocket man!
So why do we go faster? Sometimes to explore, sometimes to make money, and sometimes just because we want to win; case in point: the America's Cup, the world's most prestigious sailboat race.
So what happens when you take more than a century of engineering knowhow, add billionaire Larry Ellison and some of the world's best marine and aerospace engineers? What you get is not your father's sailboat.
How fast do these puppies go?
DIRK KRAMERS (Oracle Team USA): The top speeds are a little over 50 miles an hour.
DAVID POGUE: That's over twice the speed of a traditional sailboat.
DIRK KRAMERS: So, there's only one purpose. You just have to win a sailboat race, that's all.
DAVID POGUE: This is the Oracle, the United States' entry. It cost Ellison over one-hundred-million dollars, and he has hired the best crew money can buy. Australian Jimmy Spithill is the skipper.
JIMMY SPITHILL (Oracle Team USA): That high and the adrenaline and all that, it's just so addictive.
DAVID POGUE: Built almost entirely of carbon fiber, it's about the same length but four times lighter than a traditional America's Cup boat.
With just a few months to go before the race, I was invited onboard for a shakedown run. I'm one of the few non-crew members who's been allowed to sail with Spithill and his team.
And here we go. If you've been on a sailboat before, you might notice this is nothing like it. There's no galley, there's no bathroom, there's no wheelhouse. It's just pure speed.
Going faster in boats is not just about winning. For almost all of human history, every increase in nautical speed has shrunk the globe and exploded our horizons. Faster boats allowed the Phoenicians to bring the alphabet to the world, Columbus to discover America and Magellan to circle the earth.
Today, it's the speed of information that is rapidly shrinking our world—with ultrafast internet connections—and creating an economy where speed is measured in nanoseconds.
We are hardwired for speed, and whether it's pushing information to travel at the speed of light, or jumping out of a balloon to break the sound barrier, today we are smashing through limits long thought unbreakable.
But with speed comes danger. From high speed computer crashes roiling the financial markets…
NEWSCASTER (FILE FOOTAGE): This market is dropping precipitously.
DAVID POGUE: …to the high seas drama of the America's Cup, the faster we go, the harder we fall.
JIMMY SPITHILL: These boats reward pushing hard; you push too hard though, it can be catastrophic.
DAVID POGUE: There's safety everywhere. We're all harnessed to the boat, like this. We've got oxygen, got a knife, and we have bright orange sleeves, so if we go in the water, we're easy to spot.
The limit to how fast all things can go is determined by two factors. The first is energy, how much you can put into a system. Sailboats, obviously, get their energy from wind. But here, Oracle's engineers were able to smash through what you'd think would be a natural limit, the speed of the wind itself. The Oracle takes 15 miles an hour of wind and turns it into over 40 miles an hour of forward speed. And they do that by re-thinking the most basic element of a sailboat.
It might seem crazy, but this sailboat doesn't have a sail. Instead, it has a wing. This 131-foot-high carbon fiber wing is the boat's engine.
So how does this giant airfoil work?
SCOTT FERGUSON (Oracle Team USA): Hold this piece of paper to your mouth and blow. Blow along the top edge and see what happens to the piece of paper.
DAVID POGUE: Okay, whoa.
The paper is lifting for the same reason Frisbees® float and airplanes fly. In each case, wind traveling under the curved surface has a higher pressure than the wind above, creating lift. The same is true for sailboats, but the sail isn't horizontal, it's vertical. So here, the boat isn't pushed by the wind, it's pulled forward by low pressure.
Wow. In other words, it's sort of sucking you forward?
SCOTT FERGUSON: It's sucking you forward, exactly.
DAVID POGUE: To use the nautical term?
SCOTT FERGUSON: Yes.
DAVID POGUE: Traditional sails work the same way, but because they're flexible, they absorb some of the wind's energy. Boat designers have known for years that a rigid wing would be much more efficient. The problem was they were way too heavy. Carbon fiber changed that.
Bottom line: this boat doesn't sail, it flies.
The second key to speed is reducing resistance. As a boat moves through the water, it's slowed down by drag. So how do you solve that problem?
Their idea was to get this entire six-ton boat to lift out of the water and surf on a foil of carbon fiber.
KURT JORDAN (Oracle Team USA): It's an airfoil, just like a wing on an airplane. I mean, the fluid, whether it's air or water, accelerates over one surface faster than the other.
DAVID POGUE: In theory, it would rise up in the water just like an airplane through the air. But how about real life?
We should pick up speed, and if the boat goes fast enough, those foils in the water will do their thing and lift the boat out of the water.
The speed is intense. The thing lifts up out of the water, suddenly you're looking down 15 feet. It's like flying. It's like nothing you've done before.
After two years of training, the moment of truth arrives for Team Oracle. Their opponent, New Zealand, comes to the line in an equally innovative boat. In spite of all the technical breakthroughs that have gone into building the world's fastest boats, the America's Cup will come down to the people who sail them.
ANNOUNCER: The entire sailing world holds its breath, as they approach the line…
DAVID POGUE: The first team to win nine races takes home the America's Cup.
ANNOUNCER: …for the start of race number one of the 34th America's Cup. Three, two, one, go!
DAVID POGUE: With boats, I've learned that going faster is about how efficiently you can turn the energy put into the system, in this case the wind, into forward motion. With the America's Cup, the breakthroughs were in the sail and the underwater foil, but we can apply the same ideas to an even more basic form of human locomotion: running. From Pheidippides, who ran the first Greek marathon, to Roger Bannister smashing the four-minute mile, we've celebrated those who have run fastest.
Is there a way to make our mechanical systems, our bodies, more efficient and faster? To find out, I've come to Southern Methodist University, in Dallas, to have a friendly race against Don Miguel, a competitive sprinter from Trinidad. Our timekeeper: S.M.U. professor Peter Weyand.
PETER WEYAND (Southern Methodist University): Eleven flat; 18:04 for David.
DAVID POGUE: Weyand is a professor of speed. His lab is a high-tech lair, equipped with ultrafast cameras and a hundred-thousand-dollar treadmill, designed to measure the force of a runner's step.
Weyand believes that force is the key to speed, and he's about to show me why.
How fast is this?
PETER WEYAND: It's not as fast as it looks.
DAVID POGUE: This is nothing! Gun it! Come on! What do you got?
PETER WEYAND: Good!
DAVID POGUE: Wow. I'm like a human cheetah. Look at that! It's magnificent!
PETER WEYAND: Yeah, you look great in slow motion, David.
DAVID POGUE: Though I may be slow, when I looked at Don and me in slow motion, I was struck, not by the differences, by the similarities.
PETER WEYAND: So, what you can see is the time each of you spends in the air and the time that each of you takes to pick the limb up and put it back down in front of the body for the next step is about the same. And that's true, generically speaking, from Usain Bolt to little old ladies.
DAVID POGUE: Weyand has brought hundreds of runners through this lab, and it turns out that at top speed every one of them, including me, spends almost exactly the same amount of time repositioning limbs in between steps: about 0.3 seconds.
Our actual strides are happening, we're moving our legs, at the same speed?
PETER WEYAND: That's right.
DAVID POGUE: That guy and me?
PETER WEYAND: Competitive sprinter, you, a little old lady.
DAVID POGUE: Our legs all go at the same speed?
PETER WEYAND: The time to reposition the limbs is the same at the top speed of each of those runners.
DAVID POGUE: So what makes Don faster?
PETER WEYAND: What the competitive sprinters do is they hit the ground harder and hitting the ground harder makes your body move faster.
DAVID POGUE: When you put the graphs of Don's force and mine next to each other, the difference becomes clear. I'm hitting the ground with around 500 pounds of force; Don, 700.
The only part I don't understand is you're saying pushing down harder propels you forward faster, but it seems like pushing down harder would propel you up faster. I want to go that way.
PETER WEYAND: In the acceleration portion of a race, runners tend to lean forward so they can push backward, but that's a very short portion of a race.
DAVID POGUE: Weyand says after the first few steps, runners reach their maximum speed. From there, momentum keeps them moving forward. And the harder they hit the ground, the more time they spend in the air, and the more of their momentum they preserve.
PETER WEYAND: You push down on the ground with your limbs to pop back up, so you can maintain your forward momentum.
DAVID POGUE: This all seems well and good, but if force is speed, how do I increase mine?
PETER WEYAND: Typically, what the very fast people do is, (can we freeze it on the next contact?), when this foot comes down, the knees should be…in a good sprinter, those knees will be right together. If you eliminate the forward lean, get more upright, have your upper body be stiff, and then you want to smack the foot down hard.
DAVID POGUE: Upright posture, keep the lower body stiff, slam harder.
PETER WEYAND: Good! You felt a difference, didn't you?
DAVID POGUE: Yeah, I mean it felt like a totally different kind of running. I couldn't have told you if it was faster.
PETER WEYAND: The peak forces before were running at about 450 pounds per step. The peak forces after are well over 500: 525, 550. So that's a dramatic improvement in a matter of minutes, just from implementing a short set of instructions.
DAVID POGUE: Wow, that's amazing!
PETER WEYAND: If you can do that for the entire race, you should come down by at least a half a second to a second.
DAVID POGUE: Dog!
All right: body upright, rigid hips, hit the ground force down.
MAN: …set, go!
DAVID POGUE: Understanding the physics behind how we run…
PETER WEYAND: There you go: 16.7.
DAVID POGUE: I shaved two seconds!
…helped me more efficiently turn the energy from my muscles into speed.
To go faster and farther with those same muscles, you'd need a machine. From the first dugout canoe to the Daedalus, a human-powered plane, we have been building machines that try to get the most out of our engines: our bodies.
But the machine that really pushes the limits of human-powered speed is a bicycle. It's the most efficient human-powered vehicle there is. But what would you need to transform this humble means of transportation into a human-powered rocket? To find out, I went to Holland.
Hi, you must be Sebastiaan.
My guide is Delft University Human Power Team cyclist Sebastiaan Bowier.
SEBASTIAAN BOWIER (Delft University of Technology, Human Power Team): You want to go fast?
DAVID POGUE: He's invited me to go along for a ride.
SEBASTIAAN BOWIER: Come on.
DAVID POGUE: Sebastiaan keeps an eye on our speed.
SEBASTIAAN BOWIER: At the moment, we're going 20 miles an hour.
DAVID POGUE: He also has a meter to measure our power.
SEBASTIAAN BOWIER: And we're using about 350 watts.
DAVID POGUE: Three-hundred-fifty watts?
SEBASTIAAN BOWIER: Yeah.
DAVID POGUE: You make me sound like an electrical machine.
But it's true; it's the same kind of watts. The amount of power it takes to light up seven 50-watt lightbulbs is equal to the power I'm generating to go 20 miles an hour on this bicycle.
Three-hundred-fifty watts of raw TV-host-power! So: how to get more speed out of the energy I'm putting in?
I'm in the groove!
The answer is coming up fast.
I'm a rocket man! What was that?
SEBASTIAAN BOWIER: That's a recumbent.
DAVID POGUE: Recumbent?
SEBASTIAAN BOWIER: Yes.
DAVID POGUE: As in lying down?
SEBASTIAAN BOWIER: Yeah, you lay on your back, and because you are in a flat position, you have a lot less wind resistance.
DAVID POGUE: Whoa.
Almost half the wind resistance.
SEBASTIAAN BOWIER: So, at the moment, we're doing 350 watts…
DAVID POGUE: Compared to the racing bike, that 350 watts it took to go 20 miles an hour will get you…
SEBASTIAAN BOWIER: Twenty-five miles an hour.
DAVID POGUE: That's 25 percent faster with the same power.
So, that's cool. Just by making a few changes in the design, you wind up with the world's fastest bike concept.
WOUTER LION (Delft University of Technology): Yeah, except for that.
DAVID POGUE: What is that?
WOUTER LION: That's called the Velox3.
DAVID POGUE: Velox3.
The Velox3 has a singular mission: to become the world's fastest human-powered vehicle.
Sebastiaan is hoping that in a few months he can pedal it fast enough to break the record. It's a recumbent bicycle wrapped in an aerodynamic shell. Using the same 350 watts, today's top speed was 40 miles an hour, a 100 percent increase in speed over the conventional bike. And, believe it or not, that's nowhere near top speed.
SEBASTIAAN BOWIER: I was really easy peddling, because there is a lot of wind, so I didn't want to push it.
DAVID POGUE: That was easy peddling?
SEBASTIAAN BOWIER: Yeah.
DAVID POGUE: Sebastiaan's all-out power is 800 watts, which means he should be able to go…
WOUTER LION: Eighty miles an hour.
DAVID POGUE: Eighty miles an hour for a bike?
WOUTER LION: About, yeah it's about 80 miles an hour. That's, that's the goal of course.
DAVID POGUE: As a goal, sure, but 80 miles an hour on a bicycle? That would be more than twice as fast as the fastest Tour de France bike.
They took me into the Delft University human-powered lab to show me how they designed the Velox3 to do just that.
WOUTER LION: There are, in total, three bikes we've built.
DAVID POGUE: Each design is refined to further reduce wind resistance. Anything that creates drag is eliminated. And I mean anything.
SEBASTIAAN BOWIER: There is no window, so it's completely dark in there.
WOUTER LION: It's way better, aerodynamically, to put a camera at the back and just see through a screen.
DAVID POGUE: This all leads me to wonder, is it really even fair to call this thing a bicycle?
SEBASTIAAN BOWIER: It still has two wheels, and you have to power it yourself, so, yeah, it's a bike.
DAVID POGUE: It's sort of more of a rocket to me. As you might imagine, when you're piloting a human-powered rocket at speeds approaching 80 miles per hour, the stakes are high. This was Sebastiaan a year ago. Amazingly, he escaped without a scratch.
Now, one year later, he's back at the exact same deserted stretch of highway, in the Nevada desert, to break the world record of 82.8 miles per hour. With time running out, he pushes off for his final run.
He does it, piloting the Velox3 to a speed of 83.1 miles an hour, a new world record!
Of course if you want speed, who needs a bike? Try a '72 Buick Skylark, 350 small-block, and get some serious internal combustion muscle.
For all its failings, gasoline power is a cheap, efficient means of transportation.
What is that, a Matchbox® car?
JOHN WAYLAND (Plasma Boy Racing): It'll match your [expletive deleted], that's what it'll do. I don't think you know what you're picking on here.
DAVID POGUE: But, as I was about to find out…
Are you challenging me?
JOHN WAYLAND: I sure am.
DAVID POGUE: If you want to get more speed and power out of a car…
JOHN WAYLAND: To the track?
DAVID POGUE: See you there.
JOHN WAYLAND: All right then.
DAVID POGUE: …you have to unplug your old ideas about what makes cars fast.
ANNOUNCER: Next up, driving a 1971 Skylark, David Pogue faces off against John Wayland, in a 1972 Datsun.
DAVID POGUE: Whoa! What's going on here? Eleven-point-eight seconds? He blew my doors off!
What is this?
JOHN WAYLAND: It's a Datsun.
DAVID POGUE: I see it's a Datsun, but what's under the hood?
JOHN WAYLAND: You know what? More important, what's in the trunk?
DAVID POGUE: Oh my, what is it? A, a flux capacitor from Back to the Future?
JOHN WAYLAND: That is a lithium polymer battery pack.
DAVID POGUE: This is an electric car?
JOHN WAYLAND: You didn't know that?
DAVID POGUE: We're not talking about a Prius here. It may look like a 1972 Datsun, but underneath this humble exterior lurks the White Zombie, which holds the record for the world's quickest street-legal electric car.
So where is, like, the pistons and the carburetor?
JOHN WAYLAND: Pistons? There are no pistons; there is no carburetor. There is a controller, and there's an electric motor and there's a battery pack. It's that simple.
DAVID POGUE: That's the entire system?
JOHN WAYLAND: Yeah. It generates 538 horsepower.
DAVID POGUE: Now that's 50 percent more horsepower than my muscle car, but it's not the ponies that make the Zombie so quick.
JOHN WAYLAND: So, it's all about acceleration. And at the drag track, to accelerate, you need torque.
DAVID POGUE: Wait. So what do you mean by torque? I thought that's one of those things that's a, a spoon and a fork combined.
JOHN WAYLAND: Torque is turning force. It's the amount of energy you need to open a jar or turn a wrench. In the case of cars, it's the amount of force that's applied by the engine to the axle, to turn the car's wheels. It's usually measured in a unit called foot-pounds.
DAVID POGUE: And how many does this car get?
JOHN WAYLAND: 1250.
DAVID POGUE: That's more than a Dodge Viper and this souped-up Porsche, combined; more than any car on the road, ever.
So if this was stopped at a stoplight…
JOHN WAYLAND: Mm-hmm.
DAVID POGUE: …and you had the most powerful gas car in the world next to you…
JOHN WAYLAND: That would be a Bugatti Veyron, about a $2,000,000 car.
DAVID POGUE: …and the light turns green, this…
JOHN WAYLAND: The Bugatti would be looking at our tail lights instantly.
DAVID POGUE: Believe it or not, the hard part wasn't building a motor that powerful, it was figuring out a way to power it. In the case of the Zombie, to go from zero to 60 in 1.8 seconds, Wayland needed serious power, more than 50 Prius batteries' worth.
So how did he get that kind of juice? It took over 15 years of trial and error, dead-ends and false starts, until the answer came to him from the sky.
The batteries that start these Apache helicopters are some of the most the powerful and efficient around. They use lithium, a highly reactive element, which means it can store a lot of energy: six times as much, per ounce, as the lead in your car's batteries. Problem is, lithium-based batteries store so much energy that they can also get really hot and start fires. The solution was to combine it with manganese, which generates much less heat.
Wayland re-configured them to power the Zombie.
JOHN WAYLAND: There are 12 actual batteries.
DAVID POGUE: And not only do they allow him to go really fast, they also allow him to go farther on a charge.
JOHN WAYLAND: So, all of a sudden, my little car here, instead of just being quick and fast, we're going 100 miles on a charge.
DAVID POGUE: Okay, I'm sold. How much do you want for this?
JOHN WAYLAND: Oh, no, it's not for sale. Would you like to drive it though?
Okay. It's going to peel your face back a little bit. Your vision may get a little blurry at first, 'cause the blood runs out of your retinas.
DAVID POGUE: Oh, man!
JOHN WAYLAND: It's kind of like being shot out of a cannon.
You'll be fine, man.
DAVID POGUE: Thanks a lot. I think.
Wahoo! Yeah!
JOHN WAYLAND: Sucking amps, man!
DAVID POGUE: Sucking amps!
JOHN WAYLAND: Yeah!
DAVID POGUE: So far the breakthroughs I've discovered in making stuff faster have been tied to the speedometer, finding ways to cover more distance in less time. But there's a whole other breed of speed: it gets you there faster, not because you're breaking a speed limit, but because you're breaking a time limit.
It's called operations research or optimization, figuring out the fastest way to complete a task. It got its start in 1840, after Charles Babbage, the father of the computer, helped the British postal service figure out the most efficient way to deliver mail. Back then, scientists used slide rules to solve these kinds of problems; today they use supercomputers. So, you would think that it would be pretty easy to come up with a solution.
Well, it turns out that problems like this are still way too big for even the fastest computers. For example, what is the most efficient way to deliver 16 million packages a day? That's the problem that Jack Levis and his team at U.P.S. are trying to solve.
To understand what they're up against, let's pretend I have an Aunt Gertie, and I want to send a package to her. Its journey begins in San Francisco, Tuesday night at 8 p.m. By 2 a.m., Wednesday morning it lands in Philadelphia, only 140 miles from Aunt Gertie. Seven a.m.: it arrives here, at the U.P.S. shipping center in Gettysburg, Pennsylvania. It's already traveled over 2,500 miles in less than 12 hours. It would seem like the hard part's done, but, as I found out, it's just the beginning. Once it's on the truck, figuring out the fastest way to get it the final four miles to Aunt Gertie's, that's the real challenge. To do that, you have to solve a very difficult and complex math problem.
JACK LEVIS (United Parcel Service): The traveling salesman problem.
DAVID POGUE: Traveling salesman problem?
JACK LEVIS: The traveling salesman problem.
DAVID POGUE: What's that?
JACK LEVIS: It poses the question of what's the most efficient way to visit locations, just like a traveling salesman used to have to figure out.
DAVID POGUE: Simple right? Turns out that this problem has vexed generations of mathematicians.
JACK LEVIS: Let's say you have an office on Main Street, and you have three customers: one's on Elm, one's on High and one's on Maple. You know the time and distance between them, but what's the most efficient way to visit these customers?
DAVID POGUE: So, Elm, High, Maple, that's one. Maple, High, Elm, that's two. High, Elm, Maple: three; High, Maple, Elm: four. Four.
JACK LEVIS: Well, there's six. You know how I know there's six?
DAVID POGUE: How?
JACK LEVIS: With three customers, it's three times two, times one. There's six ways. If you double that to six customers, now there's 720 ways. At twelve customers, there's 479,000,000 ways.
If you have 25 locations to go through, there's fifteen-and-a-half-trillion-trillion different ways to visit 25 locations.
DAVID POGUE: Trillion-trillion?
So if a 25-stop route has trillions and trillions of possible combinations, imagine how many possibilities there are in the 140-stop route that will take my package to Aunt Gertie.
JACK LEVIS: There's not even a number to articulate this. There's more ways to deliver this route than the number of nanoseconds the earth has been in existence.
DAVID POGUE: So, if the traveling salesman problem is too tough for the world's largest computer, how do you solve it? By building a smarter program. It's called Orion.
How does it actually calculate the route?
JACK LEVIS: Well, it's going to start with something that's just very simplistic. It's not going to think like you, thinking ahead. It's going to say, "Who's my nearest neighbor?" So it's going to start at the beginning. "What's closest to me?" It might go to the next stop closest. So, when it's done, in its very first iteration, it's created a route that looks like a kindergartener would have created. And then it says, "Now, what's wrong with that?"
DAVID POGUE: In this case, it does that by breaking the task down into sets of smaller problems, each with five variables with 120 possible solutions. The first step is starting with clusters of five stops and figuring out the most efficient way to deliver packages within that cluster. It arranges them into larger groups of five and figures out the most efficient way to move between them. Finally, it connects them all together and arrives at a solution, with only thousands of calculations, total. And it does all this in a few seconds. But that's just the start.
JACK LEVIS: We have 8:30s; we've got 10:30s; we've got noons.
DAVID POGUE: Deliveries promised by a certain time; throw those into the mix, and it makes finding a solution nearly infinitely more difficult.
JACK LEVIS: Now you try to do that on your own and think of the number of combinations you would have to think through.
DAVID POGUE: Now here's the thing, Orion is not looking for the perfect solution, it's looking for an optimal solution that can be figured out in a few seconds. The question: is this better than what a human without a time limit could come up with?
With human pride on the line, I decided to pit man versus machine and see if I could come up with a quicker way to get to Aunt Gertie's house.
All right, so how do I compare with Orion?
JACK LEVIS: The only way to know is to give it a try. You ready to "brown up?"
DAVID POGUE: Excuse me?
Before I do my own route…
Permission to come aboard sir?
...I have to do a little boot camp. I ride with veteran driver Tim Ahn.
TIM AHN (United Parcel Service): I've done it for close to 10 years.
DAVID POGUE: Tim says it's not just about planning the fastest route, it's about how fast you can get that route done.
TIM AHN: Perpetual motion.
DAVID POGUE: Multi-tasking.
TIM AHN: Okay, I've got to move along here.
DAVID POGUE: Have you ever delivered a baby or…?
TIM AHN: I've never done that.
DAVID POGUE: I'm ready to put my traveling salesman solution to the test.
JACK LEVIS: You've got four next-day-air packages that have got to be done by 10:30. This one by 3 p.m. You got business customers that need to be delivered earlier part of the day.
DAVID POGUE: Okay.
JACK LEVIS: Pick-ups, they can set their watch by the time we get there.
Good luck. That's logistics buddy.
DAVID POGUE: The challenge here: me driving my route and Tim driving Orion's.
U.P.S.
JACK LEVIS: It's 10:30. Tim's completed 28 stops, which is pretty good. David's only got 21 stops off by now. Twelve-thirty: Tim's got 48 stops done, and he's on lunch. David's actually catching up, he's gotten 54 stops done by now, but he hasn't even taken his break. He's got to start that hour break right now.
TIM AHN: U.P.S.
JACK LEVIS: It's 2:30, and, you know, they're neck and neck right now. Tim's delivered 73 stops and David's at stop 74. So, they're neck and neck, but David still has some businesses he hasn't delivered yet. Four-thirty: Tim's now moving well ahead of David. You know, those businesses are going to close in the next hour.
DAVID POGUE: U.P.S.
JACK LEVIS: It's 6:28, and Tim is back in the building. David's got 10 more stops to do.
DAVID POGUE: Surprise!
AUNT GERTIE: Oh, my gosh! What have you been up to?
Oh, my goodness. It looks exactly like you.
DAVID POGUE: Oh, thank you.
AUNT GERTIE: Oh, I love it!
It's been such a long time.
DAVID POGUE: Yes, it has. Now, could you just please sign here? Great. See you.
AUNT GERTIE: You bet.
JACK LEVIS: It's 7:04, and David is just now coming in, 25 or 30 minutes later than Tim.
We've got to talk.
For a first-timer, looking at an as-a-crow-flies solution, it's really pretty good, but you are about 10 miles over our Orion solution.
If every driver just drove one additional mile per day, after a year, that'd be 30-million dollars. So, 10 miles for us…
DAVID POGUE: Three-hundred-million dollars.
JACK LEVIS: ...is a lot.
DAVID POGUE: And it only gets worse. My route took 39 minutes longer than Orion's to complete. Company-wide, that would be a loss of millions of hours a year.
JACK LEVIS: We'd be out of business. I'm sorry, David, but I need the browns back.
DAVID POGUE: You're firing me?
Well, I'd had a good run, but I was no match for Orion, with a plan that would, at best, bankrupt one of the largest shipping companies in the world. It was time for me to move on.
From getting packages there on the ground, to getting people there by air, these kinds of problems are everywhere you look. Case in point: did you ever feel like you spent more time boarding a plane than actually flying in it?
Oh, man, there's got to be a faster way to load an airplane!
JASON STEFFEN: You know, there is a faster way to load an airplane.
DAVID POGUE: Who are you?
JASON STEFFEN: I'm Jason Steffen.
DAVID POGUE: You've spent some time thinking about how to load a plane, have you?
JASON STEFFEN: Yeah, I wrote a computer model, ran some simulations.
DAVID POGUE: Are you, like, a computer scientist or something?
JASON STEFFEN: No, I'm an astrophysicist.
DAVID POGUE: So how does an astrophysicist from Fermilab get so fired up about finding a faster way to board a plane?
GIRL: Don't touch me!
DAVID POGUE: By getting stuck in line waiting for one.
JASON STEFFEN: I just thought to myself, there's got to be a better way to do this.
DAVID POGUE: Not a difficult problem, or so he thought.
JASON STEFFEN: The answer was obvious, that you should board from the back of the plane to the front. And so, I wrote a piece of software. When I ran it, turned out that it wasn't the best way. It seemed to be exactly the same as what I thought the worst way was, which would be boarding from the front of the plane to the back.
DAVID POGUE: Out of ideas, he was overwhelmed by the sheer number of possible plans he might have to try before arriving at the solution.
JASON STEFFEN: The number of possible ways of boarding an airplane with a hundred-and-fifty passengers would be, like, a hundred-and-fifty factorial.
DAVID POGUE: You remember factorials.
JASON STEFFEN: That's 150 times 149 times 148 times 147.
DAVID POGUE: Just like U.P.S. and the packages, this was too big a problem for a computer to handle.
So what did you do?
JASON STEFFEN: I did kind of a Monte Carlo-type simulation.
DAVID POGUE: What's a Monte Carlo simulation?
JASON STEFFEN: Just like a casino with gambling, and Monte Carlo, you basically roll the dice.
DAVID POGUE: Over and over again, each time asking a question.
JASON STEFFEN: Does this change make an improvement? If so, then I'll accept it; if not, then I won't accept it.
DAVID POGUE: In this case, instead of random rolls of the dice, he ran random plane-boarding simulations, each time keeping the faster and throwing out the slower. Through this process of elimination, the computer came up with what Jason thinks is the best solution.
JASON STEFFEN: The way my method works is the first wave of passengers are going sit in the even numbered rows, every other row, in the window seats. And then you proceed to the odd numbered rows, in the window seats. Then to the middle seats, then to the aisle seats.
DAVID POGUE: But there's a catch. In order for Steffen's plan to work, the passengers have to board in 16 different groups. If they don't precisely follow the sequence, the system will break down.
DOUG LAWSON: Yeah, well, I don't think so. There's no way that's going to work.
DAVID POGUE: And who are you?
DOUG LAWSON: I'm Doug Lawson, paleontologist.
DAVID POGUE: A paleontologist? We're talking about boarding airplanes here!
DOUG LAWSON: Well, I actually work for Southwest Airlines.
DAVID POGUE: He really is a paleontologist, and he does work for Southwest Airlines.
On the first count, he discovered one of the largest creatures ever to fly the skies. On the second, he makes planes board faster.
And what is his plan?
DOUG LAWSON: Well, what we do is just let people get on and fill the plane up.
DAVID POGUE: Southwest has no assigned seats. It just has passengers line up, board and sit where they want.
Sounds like chaos.
DOUG LAWSON: Oh, yeah, it seems like that, but people interact with one another, and we just set it up so that we get the benefit from their behavior.
DAVID POGUE: Lawson developed this plan after running hundreds of different, and, I might add, rather unusual simulations.
DOUG LAWSON: People refer to these simulations as "ant simulations."
DAVID POGUE: Ants? But we're talking about airline passengers.
DOUG LAWSON: Airline passengers are like ants in that they all have an individual job: they've got to get to a seat. And you watch what emerges as those individuals interact.
DAVID POGUE: What emerges, he says, is order rather than chaos and, most importantly, speed.
It's a shame we can't compare the two systems.
DOUG LAWSON: Yeah, well, we can! We've got a line of people waiting to get on a plane, right? Let's do a couple of experiments.
DAVID POGUE: We're going to have a battle? A battle of the plane boarding systems?
DOUG LAWSON: Right.
DAVID POGUE: Awesome!
What we have here is a Southwest Airlines 737, with which we intend to perform a historic experiment. We're going to find out, once and for all, what is the fastest way to board an airplane.
Ladies and gentlemen, welcome to the Sky Harbor International Airport Airplane Boarding Smackdown.
In this corner, from Fermilab, in Batavia, Illinois, the challenger, weighing in at 155 pounds, creator of the Monte Carlo plane boarding system, Jason "the Supernova" Steffen. And in this corner, the champion, from Niotaze, Kansas, creator of the Southwest Airlines open boarding plan, Doug "the Dinosaur" Lawson.
You know the players. You know the plans. Let's get down to business!
FLIGHT ATTENDANT: After the A group has boarded…
DAVID POGUE: First up, the Lawson open boarding plan: passengers sit wherever they like, the current industry standard for speed.
FLIGHT ATTENDANT: This is going to be a completely full flight.
DAVID POGUE: Everyone is set, queued, ready to go. And start the clock!
DOUG LAWSON: And so they'll start filling in in the front here, so you can see…
DAVID POGUE: Doug, I see we're having an overhead bin problem in 23, what's going on there?
DOUG LAWSON: Yeah, well, you know they're going to have to squish it down to try to get it in there.
DAVID POGUE: You know what they say, Doug. If it weren't for the passengers, this would be a pleasant business.
DOUG LAWSON: Right. We'd always be on time.
DAVID POGUE: Now this is going very, very smoothly, as I can see here.
Jason Steffen, I've got to imagine this is making you sweat a little bit.
JASON STEFFEN: One of the things that you run into, because people don't want to sit in the middle, is that eventually, people are going to have to start climbing over the person in the aisle seat.
DAVID POGUE: And looks like we're complete. Stop the clock! Stop the clock! Ten minutes, forty seconds, an impressive time to beat.
And we're ready for round two.
JASON STEFFEN: Tickets.
DAVID POGUE: We're going to board the same aircraft using the Jason Steffen plan.
JASON STEFFEN: That's A-52.
SOUTHWEST AIRLINES PASSENGER: Perfect.
DAVID POGUE: Steffen's plan, as you remember, is to divide folks into sixteen different groups. Have them sit in the windows first, then middles, and finally aisles. A great idea, in theory. But as we're seeing, it requires a lot of planning and direction.
SOUTHWEST AIRLINES PASSENGER: All right, thank you.
DAVID POGUE: And start the clock!
Welcome aboard.
So, if everything goes smoothly, you won't have people ever climbing over each other, or having to get out to let a middle in, because the windows will all be in first, the middles will all follow.
JASON STEFFEN: Right, and that's what's happening right now. Right now, you know, people are filing in.
DAVID POGUE: Doug, give me a critique of what might fail with this system.
DOUG LAWSON: If you can't get the window people in first, then we're going to see these jam-ups, if people were not in order.
DAVID POGUE: And sure enough, at barely two minutes in, a breakdown in the order.
JASON STEFFEN: It looks like that person,…they're not supposed to be sitting there.
DAVID POGUE: Oh, it appears, in other words, that the Steffen plan might be unraveling at the edges, just as Lawson predicted.
JASON STEFFEN: And because of that, the line's kind of backing up here a little bit.
DAVID POGUE: That should not be happening.
JASON STEFFEN: The worst that can happen is that it turns into, basically, a random boarding process all over again.
DAVID POGUE: I hate to say it, Jason, but I'm seeing a little bit of that, right now.
DOUG LAWSON: Even if you just put people on randomly…
JASON STEFFEN: They'll spread out.
DOUG LAWSON: …they would spread out.
DAVID POGUE: When you guys say it that way, it makes it sound like the system used by most of the industry, is about the dumbest possible way.
DOUG LAWSON: Yeah.
JASON STEFFEN: Yeah, that's about right.
DAVID POGUE: At six minutes in, it looks like the Steffen plan has turned a corner. The passengers seem to have finally gotten the hang of his system.
DOUG LAWSON: It's looking pretty good.
DAVID POGUE: Indeed it is, they're bolting for the window seats. Alternate rows. The aisle is being used much more efficiently because there's…
JASON STEFFEN: There's lots of people putting their luggage away at the same time.
DAVID POGUE: I must say it's going very smoothly now.
And it looks like we're complete! Stop the clock!
Seven minutes, fifty-seven seconds! A 34 percent improvement!
So while Steffen's plan won handily, by a blistering two minutes and forty-three seconds, Lawson says, because it required such a precise boarding system, in the real world, it would not fly.
DOUG LAWSON: It required so much detailed control over the whole process to get it to happen that way, and you just cannot get that kind of control in a real setting.
DAVID POGUE: And so tonight it seems that Jason Steffen may have won, but in theory, probably not in practice. What does he have to say about that?
JASON STEFFEN: I'm speechless.
DAVID POGUE: We've discovered that, in the business world, doing things faster is a science, and it can be used to squeeze out every last bit of inefficiency, saving time and making money. But what if you could move the information itself faster?
For centuries businessmen have been in an information arms race. The story is told that in the 19th century, Nathan Rothschild used a carrier pigeon to receive the news of Napoleon's defeat at Waterloo and made a fortune with that one piece of information. Today, information travels in beams of light, through fiber optic cables. That would have to be as fast as one could go, right?
Well, not so fast, this is how they used to buy and sell stocks; this is how they do it today.
This is Tradeworx headquarters, in Red Bank, New Jersey, one of the largest stock trading firms in the country. And these guys sitting at desks, they're not trading stocks, they're just babysitting the computers that do.
MANOJ NARANG (Tradeworx): Computers are doing 100 percent of the work. They're making all the decisions and they're doing all the trades.
DAVID POGUE: It's called high frequency trading. Buying and selling stocks in fractions of a second, often making less than a penny a share.
MANOJ NARANG: Average profit margin is ten percent of a cent, per share.
DAVID POGUE: But it adds up to millions, because along with speed comes volume.
MANOJ NARANG: Our firm trades over one percent of the daily market volume of the stock market—around a hundred million shares a day—so it becomes a meaningful amount.
DAVID POGUE: Right.
To make that meaningful amount, traders need to be the first to get information about prices. Speed is money.
MANOJ NARANG: In a very real way, speed is money.
DAVID POGUE: And a war has broken out between traders to get that money. The weapon: the computer networks that move financial information.
MANOJ NARANG: So, a very interesting part of the so-called "high-tech arms race" is the transmission of information across long-distance financial corridors, none of which is as important as the Chicago to New York corridor.
DAVID POGUE: Some stocks trade on exchanges in both New York and Chicago, but say a particular stock is selling for a penny less in the Chicago market. Buying that stock in Chicago, then immediately selling it in New York could turn a quick profit for whomever does it first.
MANOJ NARANG: The question is: how can you be making decisions based on the most current financial information?
DAVID POGUE: Speed has been important in the markets since the advent of markets.
DAVID POGUE: For over 150 years, buy and sell orders were transmitted over copper cable, which ran along rail lines. It took a quarter of a second for a message to complete the 2,000-mile roundtrip. And for more than a century, there wasn't any faster way, until fiber optic cable came along, in the 1980s, zig-zagging its way along the same path.
Instead of copper, fiber optic is made of glass cable, and carries messages in beams of light instead of electricity. It takes a beam of light fourteen-and-a-half milliseconds for a roundtrip on this same path, fourteen-and-a half-thousandths of a second. So how can they get faster than that?
MANOJ NARANG:Straightest possible path.
DAVID POGUE: A straight line between New York and Chicago is 720 miles.
MANOJ NARANG: But it's virtually impossible to build that route, because you have to actually go through people's property, you have to actually go through the water.
DAVID POGUE: Virtually impossible, but one of their competitors tried. It's that blue line. While they couldn't make a straight shot, their new path lopped about 175 miles off the existing one, bringing the roundtrip time down to 13.1 milliseconds. That's 1.4 thousandths of a second faster.
MANOJ NARANG: That sort of differential can translate into millions of dollars of profit or loss over the course of a year.
DAVID POGUE: Could anyone beat that?
Reluctant to leave that money on the table, Tradeworx thinks they have found a way to get even faster.
MIKE BELLER (Tradeworx): Yes!
DAVID POGUE: Tradeworx chief technology officer Mike Beller showed me what he says is the future of high speed trading. It looks something like a New Jersey used car lot.
DAVID POGUE: I think it might be a New Jersey used car lot. And next to it is our secret—or maybe not so secret—weapon in how to get from Chicago to New York faster than the next guy.
DAVID POGUE: Wow.
MIKE BELLER: Come on through here and take a look up. This is a microwave tower.
DAVID POGUE: Wait, a microwave tower?
MIKE BELLER: Yes, a microwave tower.
DAVID POGUE: Whoa, whoa, whoa!
FILM FOOTAGE: …microwave beam transmission.
DAVID POGUE: Isn't this old technology, from phone companies of the 1950s?
Even so, their plan is to set up a network of dozens of these to bounce financial information between Chicago and New York.
But I still don't understand how this can be faster than a fiber optic cable.
It's my impression that light generally travels at the speed of light.
MIKE BELLER: It does.
DAVID POGUE: So how could anything be faster than the speed of light?
MIKE BELLER: It just turns out that the speed of light in glass is a lot slower than the speed of light through the air. Light can travel this one foot of fiber in about one-and-a-half nanoseconds.
DAVID POGUE: That seems pretty fast.
MIKE BELLER: It does, but in the air, the microwave signal only takes one nanosecond to go a foot.
DAVID POGUE: What's the total time savings from here to Chicago?
MIKE BELLER: Round trip, on the order of five milliseconds.
DAVID POGUE: Shaving off those five milliseconds means they would get the roundtrip time down to eight-and-a-half-thousandths of a second. If they accomplish that, they'll gain an advantage that could make their customers tens of millions of dollars a year.
MIKE BELLER: Seems like a good deal.
DAVID POGUE: Speed is money, man.
MIKE BELLER: It sure is.
DAVID POGUE: Hey!
So, great, those guys are off making millions of dollars every millisecond with their superfast connections, while I'm waiting for the rest of the show to download.
TOM MORGAN (Polytechnic Institute of New York University): Hi, David.
DAVID POGUE: Who are you?
TOM MORGAN: I'm Tom Morgan, from Flatbush.
DAVID POGUE: Flatbush?
TOM MORGAN: Yeah, I, I'm a researcher in supercomputing at N.Y.U. Poly.
DAVID POGUE: He really is a computer scientist, and he really is from Flatbush, Brooklyn.
TOM MORGAN: You seem to be having some problems with your download.
DAVID POGUE: Well, is there anything I can do about it?
TOM MORGAN: Well, you can't build your own network, like the traders did, but you can go to Kansas City, and we can conduct a little experiment there.
DAVID POGUE: Kansas City?
Anyone home?
CHRISTOPHER BARAN (TravelingNuker): Hi. Welcome to the future.
DAVID POGUE: The future? This looks like a frat house filled with pizza-eating geeks.
CHRISTOPHER BARAN: You're in one of the homes of the Kansas City Startup Village.
DAVID POGUE: …the next generation of venture capitalists, software designers, artists and engineers.
CHRISTOPHER BARAN: So, I heard you were having some trouble trying to download your show.
DAVID POGUE: Okay, how does everybody know this?
CHRISTOPHER BARAN: Seeing the little buffer thing?
DAVID POGUE: Yes.
CHRISTOPHER BARAN: That'll never happen.
DAVID POGUE: They've all moved to Kansas City, because that's where the speed is.
CHRISTOPHER BARAN: The fastest internet connection that you can get in the United States, right now.
DAVID POGUE: And how fast is fast?
CHRISTOPHER BARAN: About 200 times faster than the average connection.
DAVID POGUE: 200 times!
The connection I was using to download the show was 5 megabits per second.
Okay, and how fast is yours?
CHRISTOPHER BARAN: One thousand.
DAVID POGUE: So where does this speed come from?
Internet giant Google has come to town and is wiring the city with…
CARLOS CASAS (Google Fiber): Fiber optic cables.
DAVID POGUE: Haven't we just been through this?
Have you considered microwaves?
CARLOS CASAS: Right now, what we have is strands of glass that transmit light.
DAVID POGUE: Yeah, fiber optic cable. The nation's internet runs on something called a backbone: a network that moves data all around the country. Now, the backbone is fiber optic cable that runs underground and on telephone poles.
CARLOS CASAS: But then they'll use copper from the pole to the home, which, really, you know, slows down the connectivity.
DAVID POGUE: That's because torrents of data, flowing at lightning speeds over fiber, slow to a trickle when they hit that old copper line running into your house.
CARLOS CASAS: What we do with our service is we are actually bringing a strand of fiber directly into a person's home. Which allows us to transmit speeds at much faster than what they're used to.
DAVID POGUE: Now this kind of speed does not come cheap; it costs upwards of $500 to wire the average house with fiber optic. But the hope is that as other internet providers follow—and some already have—the price will drop.
And what kind of difference would that make to my everyday life?
TOM MORGAN: Hi, David.
DAVID POGUE: Hi, Tom.
TOM MORGAN: How's Kansas City?
You know, David, you were having trouble downloading your TV show. Maybe we can have just a little race.
DAVID POGUE: Fastest internet in the country here, against your pathetic Brooklyn internet.
TOM MORGAN: I'm ready any time.
CHRISTOPHER BARAN: Three, two, one, go!
DAVID POGUE: Whether it's pushing the speed that information travels over the internet, or attempting a world speed record on a remote desert highway, or sailing faster than the wind…
ANNOUNCER: Race Number One of America's Cup.
DAVID POGUE: …speed isn't just about pushing the limits of our bodies; it's about pushing the limits of our ingenuity.
Thirty seconds left. What do you got remaining?
TOM MORGAN: Forty-five minutes.
DAVID POGUE: One thing is for certain…
CARLOS CASAS: Speed tends to drive innovation.
DAVID POGUE: Speed is transforming our world.
VIDEO: …a relatively unknown communication system called "the internet."
CARLOS CASAS: When we went from dialup to broadband, all of a sudden, social media, even Google became possible.
VIDEO: Over 25-million people will be using it by the year 1998.
DAVID POGUE: In less than a decade, we've come to take for granted the access to information, art and commerce that high-speed connections make possible.
But it's just the start…
CHRISTOPHER BARAN: There are some things that are going to happen that we can't even imagine.
DAVID POGUE: …because, at its best…
VIDEO: Modern equipment speeds up the world.
DAVID POGUE: …technology intended only to make things faster…
VIDEO: …a highly sophisticated computer.
DAVID POGUE: …changes the way we live our lives.
CHRISTOPHER BARAN: Those were foreign concepts. Now, with the internet, there's some things that we're going to have happen that we don't even know exist yet.
DAVID POGUE: Four, three, two, one…we are done! How are you doing, Tom?
TOM MORGAN: Oh, about, oh, 44 minutes.
DAVID POGUE: But speed alone won't get us there; we still have to chart the course.
U.P.S. Surprise!
DAVID POGUE: I haven't seen you in years!
DAVID POGUE: It was Sebastiaan Bowier's skill as a rider that propelled him to a world record. In the America's Cup, the Oracle pulled off one of the greatest comebacks in sports history.
ANNOUNCER: Emirates Team New Zealand eight wins to one. They were sitting on match point.
DAVID POGUE: After losing eight of the first nine races, the sailors of the American team fought their way back.
ANNOUNCER: And it is Oracle Team USA that is just moments away from keeping the Cup.
DAVID POGUE: Proving, once again, that no matter how fast the boat, it's still the sailor who wins the race.
We can't know where faster will take us, but one thing seems certain: since our quest for speed is fueled by our curiosity, we will continue making stuff faster.
Broadcast Credits
- Host
- David Pogue
- WRITTEN BY
- Michael Bicks
- DIRECTED AND PRODUCED BY
- Michael Bicks
Vincent Liota
Anna Lee Strachan - EXECUTIVE PRODUCER
- Chris Schmidt
- ASSOCIATE PRODUCER
- Karinna Sjo-Gaber
- EDITED BY
- Daniel Gaucher
Vincent Liota - DIRECTOR OF PHOTOGRAPHY
- Jason Longo
- ADDITIONAL CAMERA
- Stephen DeRespino
Dan Dominy
Jon Gay - SOUND RECORDISTS
- Neil Bontrager
Michael Boyle
Steve Clack
Peter Deutscher
Rusty Duggan
Michael Haines
Hugh Scott
Tyler Stephens
Willem de Wijs - DIGITAL IMAGING TECHNICIAN
- Leonardo Reyes
- MAKE UP
- Donatelle Mascari
- MUSIC
- Christopher Rife
- ART DIRECTOR
- Ekin Akalin
- ANIMATION
- Vincent Liota
Handcranked Productions
Smash5 Studios
Doug Quade - ADDITIONAL EDITING
- Max Powers
- ASSISTANT EDITOR
- Jim Fetela
- ONLINE EDITOR AND COLORIST
- Michael H. Amundson
- AUDIO MIX
- Heart Punch Studio, Inc.
- RESEARCH
- Shraddha Chakradhar
Barbara Moran - PRODUCTION ASSISTANT
- Devin McCloskey
- ASSISTANTS TO DAVID POGUE
- Jan Carpenter
Cindy Love - ARCHIVAL MATERIAL
- Corbis
Getty
Google Fiber
Human Power Team, Delft University of Technology
iStock
Johanna Lee Productions LLC
John-Michael Bills
Michael W. Sullivan
ORACLE TEAM USA
UPS - SPECIAL THANKS
- Lori Cooper
Mike Miller
Portland International Raceway
Southwest Airlines
UPS - ADVISORS
- Jim Collins
Tony DeRose
Dale Dougherty
Alex King
Tim McGee
Amy Moll
Mike Petrich
Ainissa Ramirez
Thea Sahr
Eric Siegel
Stephen Streiffer
Desney Tan
Jessika Trancik
Thomas Tretter
Phil Weilerstein - NOVA SERIES GRAPHICS
- yU + co.
- NOVA THEME MUSIC
- Walter Werzowa
John Luker
Musikvergnuegen, Inc. - ADDITIONAL NOVA THEME MUSIC
- Ray Loring
Rob Morsberger - CLOSED CAPTIONING
- The Caption Center
- PUBLICITY
- Eileen Campion
Victoria Louie - SENIOR RESEARCHER
- Kate Becker
- NOVA ADMINISTRATOR
- Kristen Sommerhalter
- PRODUCTION COORDINATOR
- Linda Callahan
- PARALEGAL
- Sarah Erlandson
- TALENT RELATIONS
- Scott Kardel, Esq. Janice Flood
- LEGAL COUNSEL
- Susan Rosen
- DIRECTOR OF EDUCATION
- Rachel Connolly
- DIGITAL MANAGING PRODUCER
- Kristine Allington
- SENIOR DIGITAL EDITOR
- Tim De Chant
- DIRECTOR OF NEW MEDIA
- Lauren Aguirre
- DEVELOPMENT ASSOCIATE
- Lisa Leombruni
- UNIT MANAGER
- Ariam McCrary
- PRODUCTION MANAGER
- Stephanie Mills
- POST PRODUCTION ASSISTANT
- Brittany Flynn
- POST PRODUCTION EDITOR
- Rebecca Nieto
- POST PRODUCTION MANAGER
- Nathan Gunner
- COMPLIANCE MANAGER
- Linzy Emery
- BUSINESS MANAGER
- Elizabeth Benjes
- DEVELOPMENT PRODUCER
- David Condon
- PROJECT DIRECTOR
- Pamela Rosenstein
- COORDINATING PRODUCER
- Laurie Cahalane
- SENIOR SCIENCE EDITOR
- Evan Hadingham
- SENIOR PRODUCER
- Julia Cort
- SENIOR SERIES PRODUCER
- Melanie Wallace
- MANAGING DIRECTOR
- Alan Ritsko
- SENIOR EXECUTIVE PRODUCER
- Paula S. Apsell
A NOVA Production by Little Bay Pictures, LLC for WGBH
© 2013 WGBH Educational Foundation
All rights reserved
This program was produced by WGBH, which is solely responsible for its content.
Image:
- Image credit: (David Pogue)
- Courtesy David Pogue
Participants
- Christopher Baran
- TravelingNuker
- Mike Beller
- Tradeworx
- Sebastiaan Bowier
- Human Power Team
- Carlos Casas
- Google Fiber
- Scott Ferguson
- Oracle Team USA
- Kurt Jordan
- Oracle Team USA
- Dirk Kramers
- Oracle Team USA
- Doug Lawson
- Southwest Airlines
- Jack Levis
- UPS
- Wouter Lion
- Delft University of Technology
- Tom Morgan
- Polytechnic Institute of NYU
- Manoj Narang
- Tradeworx
- Jason Steffen
- Fermilab
- John Wayland
- Plasma Boy Racing
- Peter Weyand
- Southern Methodist University
Preview | 00:30
Full Program
Notice: Undefined index: video_length in /home/nova/zend/application/templates/resources/multi-ep-pro.phtml on line 500
Full program available for streaming through ...
Watch Online
Full program available
Soon
Making Stuff Wilder
Host David Pogue travels the globe to explore new technologies inspired by nature. Airing October 23, 2013 at 9 pm on PBS Aired October 23, 2013 on PBS
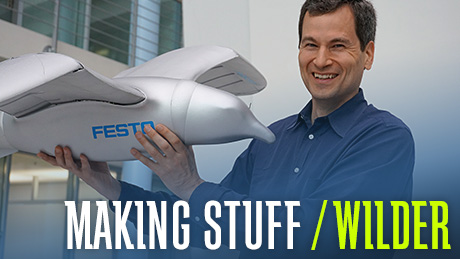
Program Description
Transcript
Making Stuff Wilder
PBS Airdate: October 24, 2013
DAVID POGUE: Civilization is built on the human drive to invent. We take the raw stuff of our planet, the materials that give names to the ages, and craft them into new forms, expanding our horizons, exploring hidden worlds and engineering life-changing technologies, always pushing the limits to be colder, faster, safer, wilder!
And now a new era is upon us, as scientists turn for inspiration to the ultimate inventor and engineer, nature. What can we learn from living things to make our own technology even better?
How long before they become self-aware and turn on their overlords?
I'm David Pogue, and I am on a quest for the world's wildest new stuff. From a carnivorous tropical plant,…
Whoa, Little Shop of Horrors.
…to an elephant's trunk,…
A little elephant snot for you.
…there's a revolution underway, as scientists borrow the best ideas nature has to offer…
I feel like an outtake from Ghostbusters.
…and put them to work, creating a robot as big as an ox,…
LS3, get up.
…and just as sturdy on its feet;…
The power!
…even teaching viruses…
I got…aw…
…to make batteries!
Come on!
Nature has been making stuff for billions of years. What happens when scientists open up its toolbox to make stuff wilder?
We humans love to invent. We've been doing it for thousands of years, but how many of our inventions really stand the test of time?
Now,imagine a world filled with only the very best stuff: amazing ideas and astonishing designs, each one the result, not just of years, not of decades, but millions of years of research and testing in an environment where the competition can be ruthless.
Since life began on Earth, it has been innovating, making discoveries in materials and engineering we've only recently begun to appreciate. The hard-won lessons of life on Earth, gained over eons, may help solve our very human problems.
Can forms found in nature reshape our machines, making them more useful? Can we build the agile movements of animals into our robots? Have some of the best ideas for new materials already been discovered by nature? What if we could make things like nature does? Can we grow the electronics and fuels of tomorrow, using the code of life itself: D.N.A.?
The search for answers to these questions has taken some strange turns.
Here at the University of Guelph, about an hour outside Toronto, materials scientist Atsuko Negishi and biologist Julia Herr think that these lovely creatures called hagfish may revolutionize how we make strong materials.
JULIA HERR (University of Guelph): So these are Pacific hagfish. They are well-known for their unique defense mechanism.
DAVID POGUE: So, if I wanted to see this, what would we do? Like, could we poke it with a stick?
JULIA HERR: I think the best way to do it is to reach in there and grab one.
DAVID POGUE: Oh, my gosh. Look at that. Disgusting.
JULIA HERR: There you go.
DAVID POGUE: Oh, no. I've been slimed! I feel like an outtake from Ghostbusters. Look at the quantities of this stuff. This is like three times the volume of the fish. How could all this slime come out of that tiny thing?
It's an impressive display of "slime-ocity." And it works great as a defense. When a gill-breathing predator bites down on a hagfish, it gets a mouth full of slime. With its gills clogged, it becomes more worried about suffocating than eating.
So how does the hagfish conjure all that slime? One of the key components is mucin, a family of proteins that includes, you guessed it, human mucus.
Mucin consists of a protein backbone, with lots of sugar side chains hanging off it, like bristles on a brush. These side chains attract water molecules, soaking them up remarkably well, in fact amazingly well.
Atsuko offers to show me what a little dab of hagfish juice can do.
ATSUKO NEGISHI (University of Guelph): So, this is a beaker of seawater. So, we're going to make some slime.
DAVID POGUE: It's getting a little misty.
ATSUKO NEGISHI: I'm just going to mix it up a little bit.
DAVID POGUE: All right.
ATSUKO NEGISHI: And if you could lift that out.
DAVID POGUE: Look at that. Oh, my gosh, that tiny dab…. Hey, there's no water left. It's taken the entire thing of water with it. That little tiny pea's worth of white stuff…
Would anyone like some? Children, there's plenty to go around.
While the mucin sucks up the water, it's a second component that holds the slime together, so I can pick it up: these threads, visible here.
Both components start out inside pores along the side of the hagfish. The water-loving mucin molecules are packed into one type of specialized cell, while the threads are wound tightly in another kind of cell. When under attack, the hagfish ejects the cells and they break open. The mucin molecules collect water molecules, while the threads, each about six-inches-long, unfurl, binding the mucin into a continuous and disgusting mass of slime.
It's these spider-silk-like threads that have really caught the researchers' attention. They might even serve as a model for a new kind of fiber, because they're surprisingly strong: ten times stronger than nylon, a synthetic material made from petroleum. If we could use hagfish fabric instead, it could help reduce our dependence on oil.
So, what are the steps involved in going from hagfish slime to handsome garments made of it?
ATSUKO NEGISHI: So, the first step would be to be able to artificially make these hagfish slime threads.
DAVID POGUE: It's early days, but Atsuko has been working on a process to create her own fiber, using proteins she's derived from freeze-dried hagfish thread. She mixes the proteins with formic acid and puts a few drops onto a salt solution, then draws up the material to create her own artificial hagfish thread.
So far, it doesn't test as strong as the original, but she has high hopes.
So you started with hagfish fiber. You treated it to come up with this component goop, put it back into saltwater, turned it back into a piece of thread. So you start with a thread, you ended with thread. Why didn't you just use the thread to begin with?
ATSUKO NEGISHI: One of the reasons is because we can't farm hagfish, and so…
DAVID POGUE: You can't farm hagfish?
ATSUKO NEGISHI: They don't currently reproduce in captivity, and so we can't have these big farms of hagfish.
DAVID POGUE: I see. Plus, it'd be a pain to get up at 4:00 in the morning to go milk the eels.
So, all of this thread-pulling is really in anticipation of the day Atsuko can synthesize her own hagfish proteins.
There it is: actual thread made of actual reconstituted fish mucus, the dawn of the era of hagfish fabric, right there.
Hmmm, what would that be like?
Nighttime is the right time for a fabric from the deep: Hagwear. But it's look, don't touch, or the surprise will be on you! Hagwear!
All right, hagfish fabric may not yet be runway-ready, but in the right hands, nature's innovations offer clues that can literally shape the stuff we make. Built to thrive in their environments, animal bodies offer winning designs and possible solutions to our own engineering challenges. After all, feathered wings inspired our metal ones; sleek swimmers helped shape our boats. What other new solutions can be found by studying the forms of animals?
I'm in Stuttgart, Germany, at the Wilhelma Zoo. It might be the perfect place to see the future shape of technology, according to engineer Heinrich Frontzek.
So, when an engineer, like you, comes to the zoo, do you see it differently from regular visitors?
HEINRICH FRONTZEK (Festo): I think so, because we want to get inspired by the nature, and here in the zoo, they're so concentrated, the huge variety of animals, all optimized for that application. And we are thinking in application, so why should…? This is a paradise for an engineer.
DAVID POGUE: Heinrich works for an automation company, trying to improve one of the most important inventions of the 20th century, the robotic arm. It's been revolutionizing factories since the first one was introduced, in 1961, at General Motors.
But robotic arms have some problems. Just like the one on humans, the traditional robot arm consists of rigid parts, joined together, often limiting its programmable motion. They're also dangerous. Get hit by one of these, and it's lights out, so robots often end up behind protective fences, unable to work closely with humans.
The German automation company Heinrich works for, Festo, decided to reinvent the robotic arm, making it more flexible and less dangerous. Heinrich leads me to the source of the inspiration. And it turns out, maybe, the best arm is a nose.
So why would you look at an elephant's trunk and think this would help you with automation?
HEINRICH FRONTZEK: As you can see, it's so flexible and transmits a lot of force and makes it much more easier to handle things. And this is our business, handling things, pick and place, to automate factory or process, and it makes sense to look into nature and to get inspired by nature, and the elephant is an excellent ambassador for that.
DAVID POGUE: Thanks to Zella, a 47-year-old Asian elephant, I get a little first-hand experience with what an elephant packs in its trunk.
Little elephant snot for you.
An elephant trunk is an impressive multi-tool, able to slurp up water…
ZOOKEEPER: Now she collects the water.
DAVID POGUE: …and squirt it.
Breakfast is on.
It picks up food like a vacuum cleaner, manipulates objects, and it's strong. Zella can use her trunk to lift over 400 pounds.
No, no, that's my wrist.
She could crush me like a bug, couldn't she?
ZOOKEEPER: Yes, yes, sir.
DAVID POGUE: Here, have some more peanuts.
But the trunk's most impressive attribute is its amazing flexibility. It comes from having no bones, and about 40,000 muscles, arranged lengthwise and in rings. With no bones and no joints, it's about as far away from a traditional robotic arm as you can get.
Beep! Beep!
I head to Festo's headquarters, in nearby Esslingen, to see their version of the elephant trunk. They call it a "bionic handling assistant."
Now, this looks like a bionic handling assistant.
HEINRICH FRONTZEK: Yeah. You're absolutely right. This is our trunk.
DAVID POGUE: Festo's version of the trunk is made of plastic, with a series of air chambers inside. Filling different parts with compressed air causes it to bend.
So if I wanted to bend it that way?
HEINRICH FRONTZEK: We need a tube with compressed air for this expansion and then you get this bending to the other end.
DAVID POGUE: So this blows up like a balloon?
HEINRICH FRONTZEK: Yes, for sure.
DAVID POGUE: They're testing the assistant with this simple motion, for use in a packaging operation.
Look at that, it tucks it in nicely. Well done, Dumbo.
But it is inherently more flexible than a conventional arm, and, just as important, far safer.
HEINRICH FRONTZEK: We don't have electricity, we don't have steel and iron and all this masses, which are…could damage a person. It's a weight of five pounds. Some valves, a little control system…
DAVID POGUE: So there's really nothing here but plastic tubes and air.
HEINRICH FRONTZEK: Yeah.
DAVID POGUE: Does it do tricks?
In this application, the tip of the trunk works by suction, but Festo has experimented with what it calls a "fin gripper," inspired by fish fins.
If you push on the middle of a tail fin, it doesn't curl away from you as you might expect, it curls toward you, giving a fish much more efficient strokes. But Festo has built that principle into a gripper that curls around the object it needs to pick up, adapting to the shape.
HEINRICH FRONTZEK: When we have to change the shape of the products we are handling, so, we need something flexible, more flexible. For this, it is very helpful to use a fish tail as a gripper.
DAVID POGUE: So, it looks to me like you're about to demonstrate how this might work.
HEINRICH FRONTZEK: Yes. We have two different gripping devices. One with a fish tail, and this is a traditional one the robots are using.
DAVID POGUE: Can I see these things close?
HEINRICH FRONTZEK: Sure. Same pressure, everything is equal.
Now, we will see what happens. This is the old robot, and this is the bio-engineered method.
DAVID POGUE: Okay. Let the competition begin.
Look at that! Traditional robot hand, shattered to smithereens, and the fish tail gripper really did its job. So you have robot, zero; fish tail, one. You have stolen from nature and did a great job.
HEINRICH FRONTZEK: Thanks.
DAVID POGUE: Thank you very much. Oops! They'll edit all this out.
Combining the fin gripper with the elephant trunk produces a flexible, lightweight and safe robotic arm, ready for all sorts of applications.
HEINRICH FRONTZEK: Biomimicry, nowadays, it's part of the design process here at Festo, so everybody is using this cross-thinking, get inspired by nature and to transform these ideas into industrial applications.
DAVID POGUE: May I? Thank you.
Festo's handling assistant steals its form from the elephant trunk, but Festo isn't alone in adapting designs found in nature and applying to them to industry. The beak of the kingfisher bird breaks the water with very little resistance, inspiring the shape of this Japanese bullet train, so it would cut efficiently through the air. The shape of the yellow boxfish provides a rigid structure, and has very little drag for such a large volume, all reasons Mercedes-Benz used it for the design of a high-efficiency concept car.
But making machines that look like animals is one thing, what about making machines that move like them?
For thousands of years, when we've invented new forms of transportation, many have been based on a human insight not found in nature at all, the wheel. But there are plenty of places wheels can't go, even ones wearing a belt of tank tread.
The inability of our machines to traverse difficult terrain has dire consequences on the battlefield and in search and rescue. But while wheeled vehicles struggle off-road, there are some creatures getting around on legs. That's had engineers wondering, "What lessons can we learn from animal movement? Can we give our machines a leg up?"
Walking is easy for animals; even a toddler can do it.
C3PO/STAR WARS: Excuse me, sir.
DAVID POGUE: And, thanks to movies, creating walking machines seems easy, too. Just look at C3PO from Star Wars…
C3PO/STAR WARS: C3PO: Oh! Nice to see a familiar face…
C3PO/STAR WARS:
OTHER BOT: It Chuta.
C3PO/STAR WARS: C3PO: How rude!
DAVID POGUE: …or its walkers. You'd think the problem's been solved, but in real life, it's hard. One of the best-known early attempts at a walking machine is General Electric's walking truck, from the '60s. It even tackled uneven terrain, but it took a human operator to decide where to place each foot, one at a time, an exhausting task.
By the '70s, computer control automated the walking motion in a series of crawlers, built around the world, though they werestill driven by human operators. These kept a tripod of legs on the ground, maintaining stability at all times, a system called "static balance." They moved slowly, like a walking table.
But in the 1980s, a very different approach gained ground.
I've traveled to Massachusetts, to visit a company that builds robots based on that work. The company's founder, Marc Raibert, has been building walking robots for over 30 years. Early on, he steered away from the static balance of walking tables.
To help me understand how he views animal locomotion, he invites me to take a ride on a pogo stick. It's tricky, because, like all standing humans, I am top-heavy; in technical terms: an inverted pendulum.
MARC RAIBERT (Boston Dynamics): Here is a normal pendulum, right? If you swing it…
DAVID POGUE: The weight is at the bottom. Yeah.
MARC RAIBERT:But if you put the weight at the top, what happens? If you don't do anything, it tips over, but if you move the point of support, you can keep it balanced.
DAVID POGUE: If you're top-heavy, staying balanced requires keeping your base of support under your center of gravity. That's what Marc is doing by shifting the bottom of the broom as it tips; that's what I'm doing by moving around the pogo stick, and that's what all of us do, all the time, when we're upright.
In fact, the human brain receives constant updates to maintain the body's balance: from the inner ear, where a series of fluid-filled canals send signals about the position and motion of the head; from the eyes, which send signals about the body's position relative to other objects; from internal sensors that tell us about the position of body parts relative to each other; and from external pressure sensors in the hands and feet, that send signals about the source of support, for example, if you're on uneven ground. All of this information feeds into our cerebellum, which keeps our top-heavy body from tipping over, even when we're just standing around.
Without it, you would topple over.
To Marc, we are less like a static table and more like a pogo stick. To focus on the problem, he built a robot that had only one springy leg. It constantly calculated where its weight needed to shift to stay upright, very pogo-stick like.
Even when he added more legs, he kept the bounce in their step, and an active sense of balance.
For the last few years, Marc has been applying what he's learned to solve a problem for the U.S. military. On rough terrain, wheeled vehicles aren't much use. And soldiers often haul everything on their backs, leading to injuries and exhaustion. Marc invites me to see Boston Dynamics' solution, out at a nearby park.
Meet LS3, also known as AlphaDog. It's designed specifically for rough terrain, anywhere a soldier might go on foot, and it carries 400 pounds of gear, along with enough fuel for a 20-mile mission.
MARC RAIBERT:So, Dave, in this mode, the robot is following the leader. He's got a backpack on and has some reflective stripes on it, and the vision system focuses on that and looks at them, and then it records what path he takes through the terrain.
DAVID POGUE: Is it modeled after a particular animal, an ox or a horse or…?
MARC RAIBERT:You know, not really. We take inspiration from how animals are designed and how they're implemented, but then we have to use human engineering tools, in the end, and human materials, so sometimes it stays like the animals, sometimes it departs.
DAVID POGUE: I ask Marc for a tour of LS3, of course, after it's been shut off.
MARC RAIBERT:So this is the leg, and it's got a muscle here, or the actuator, which causes it to move. This muscle moves the knee joint. The computer is really the equivalent of a laptop-style computer. So, you know, all the balancing is done in that computer. All the speed control, all the turning is done in that computer.
There is a laser range-finder here that provides 3D-depth information. There is a set of cameras here that are used to track a human leader. There's other cameras under here that look right in front of the robot and provide information about the shape of the terrain,…
DAVID POGUE: Wow.
MARC RAIBERT:…so that the feet can pick the best places to step.
The legs themselves can feel the forces that are exerted at all the actuators.
DAVID POGUE: Does it ever slip? What if it steps on an oily leaf or something?
MARC RAIBERT:It slips, and frequently it corrects for those slips. So, the goal is to make it so that the feet can slip and the control system recognizes that it's slipping and compensates by using the other legs.
DAVID POGUE: Lots of cool tech on LS3, but my favorite feature? Voice control!
Let's see. Power on, engine off, sit, get up and get me a beer. That's a good one. I like that.
LS3, get up.
The power!
LS3, follow tight.
What a good boy.
You can do it. Come on, come on!
LS3, sit. LS3, power off. I think you got something here.
Nice.
In a final test of LS3, Marc has it "follow the leader" up a steep incline. Here, you really see it actively balancing, while in motion, just like me on the pogo stick, instead of moving from one stable position to another like a walking table.
MARC RAIBERT:And the idea that you could have it passively stabilized like a table, you know, that doesn't really work with a moving robot. There is too much energy in the motion of the body. And I believe that the only way to make these things work is to really commit to the active balance.
DAVID POGUE: LS3 isn't really built for speed. It trots at about five miles an hour.
But what would it take to make it go faster? This is the Cheetah. Just like the real deal, its back flexes with each step, increasing the stride of its gallop. Right now, it is the fastest robot with legs in the world.
But start looking over your shoulder for the next generation: WildCat. It's designed to be untethered.
Four-legged robots have their uses, but events like the recent Fukushima nuclear disaster have renewed interest in the human form. Radiation kept people at bay, away from all available rescue equipment, from cars, to power tools, to shut-off valves. But imagine if there'd been an easily controlled humanoid robot to operate them?
Robotics engineers have been working on that for years. In 2009, Boston Dynamics introduced PETMAN, a robot that balanced itself, walked and even did some calisthenics.
Over the last few years, PETMAN has evolved into Atlas, which has even more mobility. Just like LS3, it actively balances itself all the time. And in this impressive demo, all by itself, it uses its arms to work its way past a hole in the floor.
Today, they're tweaking its sense of balance on one foot.
ROBERT PLAYTER (Boston Dynamics): Looking at what test to do here, we studied gymnasts. And when they are just about to fall off, you'll notice that they throw their arms and their legs around very violently. And so, we're trying to understand what techniques they're using, to build a robot that can really handle rough terrain.
DAVID POGUE: They've been doing this test for only a week. First, the robot goes up onto one foot. Then they hit it with a 20-pound medicine ball.
ROBERT PLAYTER: So, if you notice there, it's swinging its arms and legs all around, in kind of a clockwise fashion, and that momentum helps move the center of mass back over the feet, not dissimilar to a way the gymnasts do it.
DAVID POGUE: Oh, ho, ho.
Let me at it!
Now, let's see some human dynamic balancing.
ROB PLAYTER:The robot's blind. It doesn't know the ball is coming.
DAVID POGUE: Oh.
ROBERT PLAYTER: So, we don't want you to know the ball is coming either. So, we've got a little blinder there for you, so you don't see the ball coming.
DAVID POGUE: Oh, great. So, I don't know when the ball is coming.
ROB PLAYTER:That's right. Use whatever technique you think you need to stay upright.
DAVID POGUE: I have a feeling, if your stinking hunk of silicone and hydraulics can do it, I can, of course, do it, too.
ROB PLAYTER:That's right.
DAVID POGUE: Side by side, it's hard to say who does it better. The Atlas seems more stable, but I have a few other tricks up my sleeve.
MARC RAIBERT:Very good.
DAVID POGUE: I admire your robot, sir.
ROBERT PLAYTER: Well, I admire you wearing those glasses on public television.
DAVID POGUE: We'll be seeing more of this guy. Atlas is the hardware used by seven software-development teams in an international rescue-robot competition.
But a single, sophisticated and expensive robot like Atlas is just one strategy. What about a less expensive and less complex machine, but more of them?
That's the idea behind Harvard University's RoboBee. It would take 30 of these to equal the weight of a penny.
What happens when you move beyond having just one robot and, instead, have a swarm? In the future, swarms of robots operating as a team might build our skyscrapers or map uncharted areas or scout out victims in disasters, as robotic search and rescue teams.
But in order to do any of that, engineers must solve a problem nature solved eons ago: "How do you get a group of individuals to work together as one?"
In nature, swarms often behave as if they have a collective intelligence. Whether it is fish schooling in the sea, or birds flying in a flock, the members act in unison, without anyone, apparently, in charge.
Some of the achievements built out of this swarm intelligence are awe-inspiring, like this murmuration by thousands of starlings, or these complicated towers built several feet high by blind termites.
So what can we learn from behavior in nature about creating robotic swarms?
Vijay Kumar and his students at University of Pennsylvania have been wrestling with the problem. They use a fleet of hand-sized quadrotor robots, which they've learned to manipulate with impressive control. They can play the theme from James Bond or put on a lightshow. In both performances, the quadrotors are individually controlled by a central computer.
But they've also built some computing power into individual robots, so they can think for themselves, like figuring out how and when to fly through a tossed hoop.
Now, Vijay is taking the next step, developing software that will allow the bots to work together as a swarm, a team that can do more than any single flyer can.
One flying bot, pretty cool; eight flying bots? It gets a little "swarm" in here.
VIJAY KUMAR (University of Pennsylvania): So what you see here is these robots are commanded to rise into a swarm. They're asked to form patterns, three-dimensional patterns, and then the robots figure out what point in the pattern to step into and how to coordinate with their neighbors.
DAVID POGUE: Oh, so the master computer doesn't say, "You be in the corner." It's just saying, "Be a rectangle," "Be a circle," but they have to decide how to execute that?
VIJAY KUMAR:Right.
DAVID POGUE: Well, a central computer could control each of the eight robots individually, telling them where to go, but Vijay wants a system that scales up, and with more robots, no computer could keep up.
So, instead, he's taken inspiration from swarms in nature, and developed three guiding principles.
First, as much as possible, just as in nature, each robot thinks for itself. Second, each robot acts primarily on local information it gathers, the way a bird in a flying flock probably pays attention only to its immediate neighbors to know where to go. Finally, no one robot is in charge. They're all interchangeable, so that if one breaks down, the group continues.
To test out those principles, Vijay turns his fleet over to me and lets me experiment.
The flyers know they're supposed to make a circle. As I add them, one at time, you can see it take shape. Or, I can randomly pluck one out of the air—proving none is essential—and put it back somewhere else. Its neighbors adapt.
Of all the possible applications, Vijay sees a big future for swarms in search and rescue. And he shows me how it would work.
VIJAY KUMAR:So, imagine you have a victim, you can imagine robots wandering around looking for, maybe, a cell phone signal that might tell you where the victim is.
DAVID POGUE: Find that, robot drones!
BENJAMIN CHARROW (University of Pennsylvania): I'm telling these bots to move.
DAVID POGUE: The numbers of the bots?
BENJAMIN CHARROW: The numbers of the bots. They're all moving around.
DAVID POGUE: All right, there they go. They've chosen independent routes.
In this demonstration, run by one of Vijay's grad students, the robots roam the floor, measuring the strength of a signal transmitted by our lost victim's cellphone. They share their readings, creating a map.
In effect, the transmitter is saying, "Warmer, warmer."
BENJAMIN CHARROW: Cooler, cooler. But the robots know not to trust any one sensor reading, too much.
DAVID POGUE: The swarm of robots cut the overall search time, by gathering information faster than a single robot could, leading to the rescue of our lost little guy.
VIJAY KUMAR:Well, right now it's a lab study. But this sort of illustrates one of the directions in which we want to go, which is you take very simple robots with very simple sensors, so they're inexpensive, you put them together, and suddenly you have the benefits of these robots collaborating to do things that they individually cannot do. And that illustrates what swarm technology can really do for you.
DAVID POGUE: We've seen how nature has inspired the way robots look, how they move and even how they act in concert in swarms, but living things have also inspired engineers on a more fundamental level, the very stuff we make things out of, our materials.
Many of our modern materials have taken their cues from the natural world, especially plastics. Silk, the product of worms, inspired nylon, and the search for a substitute for rubber led to the invention of polystyrene, the stuff we often call STYROFOAM™.
But natural materials also hold hidden secrets, tiny structures, invisible to the naked eye, that can give them near magical properties, properties we can mimic.
For example, the tips of microscopic hairs on the feet of wall-climbing geckos have led to the creation of material for wall-climbing robots.
So the hunt is on. What other secrets might living materials reveal?
I've travelled to Harvard University to meet materials scientist Joanna Aizenberg. She's taking me to see a plant with tiny structures on its surface that play a slick trick, one we might use for new materials in sticky situations.
Whoa, creepy. Little Shop of Horrors. What is this plant?
JOANNA AIZENBERG (Harvard University): This is a Pitcher plant, a carnivorous plant. It eats stuff, eats insects.
DAVID POGUE: It's called the Pitcher plant for its pitcher-shaped leaves, though when it comes to ants and other insects, these Pitchers throw a mean curve. In dry weather, ants can easily walk on the lips of the Pitchers, using their sticky, oily feet. But in wet conditions, the ants and their oily feet get a Little Shop of Horrors surprise.
Here's how the trick works. On a dry day, the surface of the Pitcher plant looks like this, no problem for the ants. But add water and it sticks to the bumpy surface, creating a slippery wet film, a Slip 'N Slide® for ants.
JOANNA AIZENBERG: All these insects just slide into digestive juices inside the organism.
DAVID POGUE: They fall in?
JOANNA AIZENBERG: They're hydroplaning inside this plant.
DAVID POGUE: Cool.
To Joanna, the Pitcher plant's "slippery-when-wet" strategy seemed a promising start for developing new non-stick materials, but only a start.
JOANNA AIZENBERG: It evolved this structure to capture prey. It didn't evolve this structure to create these slippery materials on metals, on plastics, on glass. So this is where material scientists come in. Let's design something similar.
DAVID POGUE: Taking the Pitcher plant as inspiration, Joanna has developed a new non-stick surface treatment, a new way to keep stuff clean. She calls it "Slippery Liquid-Infused Porous Surfaces," or SLIPS.
We start with a big piece of aluminum. The right is untreated, the left has SLIPS.
So, we're going to try putting some stuff on each half, and we'll see what sticks.
JOANNA AIZENBERG: And what slips.
DAVID POGUE: Well done.
First step: chocolate sauce. Haven't I seen this on late night TV?
I hate getting chocolate on my aluminum.
PHIL: And on SLIPS.
DAVID POGUE: Look at that, it beads right off. Now how much would you pay?
Cleaning off the chocolate with water works, but not as easily as SLIPS.
By gosh, Phil, you didn't even need that water. It rolled right off.
But let's raise the stakes. Next up, motor oil.
Oh, Phil, you're ruining this perfectly good sheet of aluminum. That'll never come off.
What? It rolls right off!
JOANNA AIZENBERG: While this one leaves a stain that is not going to be removed, even with washing. It's actually getting even dirtier.
DAVID POGUE: And on the SLIPS side, no contest.
Well, I can't imagine anything much worse than motor oil.
Liquid asphalt!
If we had a studio audience, they'd be going, "Ugh!"
PHIL: And on SLIPS.
DAVID POGUE: Come on! Even tar rolls off like off a duck's back.
Seriously, it's an amazing display of "unstickiness," and it works in a totally different way than the current king of unsticky, Teflon®.
Teflon is a plastic polymer, a long chain of repeating molecules. It has a carbon backbone, with fluorine atoms tightly bonded to it on the outside. The fluorine acts as a shield, preventing the normally reactive carbon from bonding with anything else, the secret of Teflon's unstickiness.
But SLIPS works in a totally different way: on the Pitcher plant principle. First, Joanna adds a porous material, less than a hundredth of a centimeter thick, to the stuff she wants to protect, in some cases, just by spraying it on. Then she adds a liquid, often an oil, that seeps in. The layer and the liquid are formulated to attract each other, keeping the liquid in place.
Just like the water on the lip of the Pitcher plant, the liquid creates a smooth non-stick surface on top.
Joanna believes there are lots of applications. Graffiti a problem? Not on the sign that's been treated with SLIPS. SLIPS helps prevent ice buildup, by repelling water before it has time to freeze. And if ice forms, SLIPS defrosts more quickly, which may lead to applications in refrigeration and the deicing of planes. It's a clever use of biology as inspiration.
JOANNA AIZENBERG: Bio-inspired is more taking some clever solution and maybe reformulating it in a different way, using something different that nature doesn't use, but the design is preserved.
DAVID POGUE: So SLIPS would bear the label, "Based on an idea by nature."
JOANNA AIZENBERG: That's right, yeah.
DAVID POGUE: And, of course, the research continues.
Rinse. SLIPS. It's absolutely repellent.
SLIPS cleverly adapts one of nature's innovations, but, increasingly, scientists have been asking a new question: "Can we adapt, not only the stuff nature makes, but also the way nature makes stuff?"
Today's manufacturing consumes vast amounts of natural resources, while creating mountains of waste, some of which is toxic. Take paper. To make a ton of it, you start with almost twice as much wood and generate thousands of pounds of waste.
The leather industry is even worse. A ton of finished leather requires over five tons of the raw stuff.
Compare that to the natural growth of plants and animals. They take what they need to build their bodies—no blasting furnaces, no acid baths, no pounding machinery—and waste that recycles easily. What if we could recruit nature to grow the stuff we need for our technology? What if we could grow a car or a computer or a cell phone? Can we make our manufacturing more like nature's?
M.I.T. professor Angela Belcher, may have taken the first step toward realizing that dream.
ANGELA BELCHER (Massachusetts Institute of Technology): Well, I'm really interested in how nature makes materials, how organisms in the ocean have evolved to use just the elements of their environment to make really exquisite materials, like this abalone shell here.
DAVID POGUE: Angela first took inspiration from the abilities of the humble abalone, whose intricately structured shell is made in part out of calcium. Over the course of its life, the abalone takes calcium atoms from the ocean and slowly assembles them into its strong shell, a talent its ancestors likely developed several hundred million years ago, which made Angela ask a question: "If life could learn to build a shell with calcium, could it learn to build stuff using other elements, like the ones we use for our technology?"
It didn't seem likely. If you look at the Periodic Table, life primarily uses only six elements, along with a smattering of others.
On the other hand, our technologies, our computers, our electronics, rely heavily on elements that nature largely ignores. Angela wondered. Could she coax organisms into building stuff out of our high-tech elements, let's say a battery?
Batteries have three components: a negatively charged electrode, a positively charged electrode and a separator, called an electrolyte.
To test her idea, Angela decided to make a negative electrode by growing it.
But what creature would do the growing?
She sends me down to the water, promising to show me the type of organism she recruited.
I got, oh. This better be worth it.
I'm sorry, I did not actually manage to get any sea organisms in there.
ANGELA BELCHER: You did. There's actually tens of millions of viruses in the sample.
DAVID POGUE: Viruses? Is this one of those medical waste beaches?
ANGELA BELCHER: No. These are viruses that are a natural part of the environment, a really important part of the ecosystem of the ocean.
DAVID POGUE: It turns out, viruses are actually the most abundant organisms in the ocean. A teaspoon alone holds over 10-million of them. Viruses come in all kinds of shapes, but in some ways they are all the same. They're each a little bit of genetic material wrapped in a protective coating.
Scientists have learned how to change the genetic material inside some viruses, altering their behavior, making some strains into popular and safe lab tools, for research.
For her work, Angela chose one of those safe lab viruses, which happens to be long and thin, a little like a pencil. This virus reproduces by latching onto a bacterium, injecting its D.N.A., and forcing the bacterium to produce millions of copies of the pencil-shaped virus.
I have a model with me. This is what a virus looks like?
ANGELA BELCHER: Yeah, exactly like that. So I have a model here.
DAVID POGUE: A much better one, yeah?
So this is designed to stab the bacteria?
ANGELA BELCHER: That's exactly right. Like the pencils stab…
DAVID POGUE: But you're going to teach it a new trick?
ANGELA BELCHER: Right. I'm going to teach it, instead of to bind the bacteria, to grow in electronic material.
DAVID POGUE: Come on. That's like saying, "Well this is where I'm going to teach the rabbit to do microsurgery."
The virus normally sticks to the outside of the bacterium it targets, but what Angela wanted was for the virus to bond with little bits of metal. And yes, that is weird.
ANGELA BELCHER: So imagine these are tiny bits of metal that's going to be part of a battery electrode. Now, this virus has not been repurposed yet, so it has no ability to bind this material, so if there's no reason that would bind these small pieces of metal…
DAVID POGUE: The stickiness her virus has for a material is determined by the virus's outer coat, which in turn, is built by its D.N.A. So, Angela had to change the D.N.A., rearranging its A, C, G and T building blocks, to change the outer coat, so metal would stick to it.
And you have the ability to modify, mutate their D.N.A.?
ANGELA BELCHER: That's right. We're going to go in, and we're going to add D.N.A. sequences to them, but we're going to do it at random D.N.A. sequences. So, it's like rolling a bunch of dice.
DAVID POGUE: Angela used a process called "directed evolution." She randomly mixed the A, C, G and T building blocks of D.N.A. and inserted the bits into the virus, creating billions of variations.
Then, she tested them to see which viruses bonded to electrode material, until she found the one that worked best.
ANGELA BELCHER: What you get is a virus that's completely coated in that metal that makes up this battery electrode material.
DAVID POGUE: Just like an abalone assembles calcium into a shell, her virus could now assemble metal into a tiny electrode.
By packing together millions of the metal-coated viruses, Angela made a negative electrode large enough for a battery. She repeated the process to create a virus that assembled the positive electrode. Adding an off-the-shelf electrolyte, she completed her virus battery.
The virus batteries can take many shapes. These are coin batteries used in electronics. And Angela has created about a hundred other specialized viruses that make other products, including solar cells with improved performance, thanks to the virus inside, and specialized materials that enable chemical reactions, catalysts, that create fuels.
Is this all about making better batteries and solar cells, then, or is this something bigger?
ANGELA BELCHER: Well, I think it's something bigger. It's a new way of manufacturing materials. Use biology to come up with new ways of manufacturing materials that have improved performance.
DAVID POGUE: So let's compare the old way versus the new way. Old way: high temperature, piles of waste and lots of toxic byproducts; new way: room temperature, little wasted material and few toxic byproducts, with a goal of none.
As it emerges from the lab, Angela Belcher's work holds the promise of cleaner manufacturing and a new partnership between our industrial ambitions and the best manufacturer on the planet: nature itself.
Form, movement, behavior, materials, manufacturing, all ways nature has led us to seek out new solutions to our technological problems, in the amazing abilities of plants, animals and even viruses.
But while biology may already hold the answers to tomorrow's challenges, in the hard-won lessons of evolution, it still takes the creativity and hard work of engineers and scientists to recognize them, combining the best of both worlds. That's Making Stuff Wilder.
Broadcast Credits
- HOST
- David Pogue
- WRITTEN, PRODUCED AND DIRECTED BY
- Daniel McCabe
- EXECUTIVE PRODUCER
- Chris Schmidt
- ASSOCIATE PRODUCER Cara Feinberg
- EDITED BY
- Daniel McCabe
Justin Vaida - DIRECTORS OF PHOTOGRAPHY
- Jason Longo
Stephen McCarthy - ADDITIONAL CAMERA
- Richard Fox
Bruce Douglas Johnson
Thorsten Thielow - SOUND RECORDISTS
- Steve Bores
Mario Cardenas
John Garrett
John Gooch
Kim Hartmann
Michael Krikorian
Axel Lischke
Adrian Tucker - CAMERA ASSISTANT
- Gregory Brutus
- ASSISTANT EDITORS
- Elliott Choi
Jim Fetela - PRODUCTION ASSISTANT
- Tesla Cariani
- FIELD PRODUCTION ASSISTANT
- Walker Edelman
- MUSIC
- Christopher Rife
- ART DIRECTOR
- Ekin Akalin
- ANIMATION
- Smash5 Studios Handcranked Productions, LLC
- POPCORN TECHNICIAN
- Bob Burns
- ACTOR
- Maria Davis
- ONLINE EDITOR AND COLORIST
- Michael H. Amundson
- AUDIO MIX
- Heart Punch Studio, Inc
- ASSISTANTS TO DAVID POGUE
- Jan Carpenter
Cindy Love - ARCHIVAL MATERIAL
- Action Sports/ALL-STOCK
The Associated Press
Dr. Kellar Autumn
Dr. Ulrike Bauer
Corbis Motion
Dr. Mark Cutcosky
Daimler AG
Defense Video & Imagery Distribution Systems
Festo AG & Co. KG
Footage Direct.com LTD
Footage Search, Inc.
Framepool, Inc.
Getty Images
godutchbaby
KMel Robotics
Museum of Innovation and Science
Museum of New Zealand Te Papa Tongarewa
Pond5
Saatchi & Saatchi Worldwide
Kurtis Sensenig
Shutterstock, Inc.
T3Media, Inc.
University of Guelph
USA Gymnastics/NBC Sports
Sophie Windsor Clive and Liberty Smith, Islands & Rivers
The WPA Film Library - SPECIAL THANKS
- AeroVironment, Inc.
Leslie Ballard
Boston Dynamics
Jean Brandt
Department of Biological Engineering, Massachusetts Institute of Technology
Department of Organismic and Evolutionary Biology, Harvard University
Ecovative Design, LLC
The Estate of George C. Devol
EvoLogics, GmbH
Festo AG & Co. KG
GRASP Lab, University of Pennsylvania
Devin McCloskey
James McClurkin
Robert Bosch GmbH
Seacoast Science Center
Tower Hill Botanic Gardens
United Parcel Service, Inc.
University of Guelph
Jerry Weinstein
Wilhelma Zoologisch-Botanischer Garten, Stuttgart, Germany
The Wyss Institute for Biologically Inspired Engineering at Harvard University - ADVISORS
- Jim Collins
Tony DeRose
Dale Dougherty
Alex King
Tim McGee
Amy Moll
Mike Petrich
Ainissa Ramirez
Thea Sahr
Eric Siegel
Stephen Streiffer
Desney Tan
Jessika Trancik
Thomas Tretter
Phil Weilerstein - NOVA SERIES GRAPHICS
- yU + co.
- NOVA THEME MUSIC
- Walter Werzowa
John Luker
Musikvergnuegen, Inc. - ADDITIONAL NOVA THEME MUSIC
- Ray Loring
Rob Morsberger - CLOSED CAPTIONING
- The Caption Center
- PUBLICITY
- Eileen Campion
Victoria Louie - SENIOR RESEARCHER
- Kate Becker
- NOVA ADMINISTRATOR
- Kristen Sommerhalter
- PRODUCTION COORDINATOR
- Linda Callahan
- PARALEGAL
- Sarah Erlandson
- TALENT RELATIONS
- Scott Kardel, Esq. Janice Flood
- LEGAL COUNSEL
- Susan Rosen
- DIRECTOR OF EDUCATION
- Rachel Connolly
- DIGITAL MANAGING PRODUCER
- Kristine Allington
- SENIOR DIGITAL EDITOR
- Tim De Chant
- DIRECTOR OF NEW MEDIA
- Lauren Aguirre
- DEVELOPMENT ASSOCIATE
- Lisa Leombruni
- UNIT MANAGER
- Ariam McCrary
- PRODUCTION MANAGER
- Stephanie Mills
- POST PRODUCTION ASSISTANT
- Brittany Flynn
- POST PRODUCTION EDITOR
- Rebecca Nieto
- POST PRODUCTION MANAGER
- Nathan Gunner
- COMPLIANCE MANAGER
- Linzy Emery
- BUSINESS MANAGER
- Elizabeth Benjes
- DEVELOPMENT PRODUCER
- David Condon
- PROJECT DIRECTOR
- Pamela Rosenstein
- COORDINATING PRODUCER
- Laurie Cahalane
- SENIOR SCIENCE EDITOR
- Evan Hadingham
- SENIOR PRODUCER
- Julia Cort
- SENIOR SERIES PRODUCER
- Melanie Wallace
- MANAGING DIRECTOR
- Alan Ritsko
- SENIOR EXECUTIVE PRODUCER
- Paula S. Apsell
A NOVA Production by Big House Productions, Inc. for WGBH/Boston
© 2013 WGBH Educational Foundation
All rights reserved
This program was produced by WGBH, which is solely responsible for its content.
Image:
- Image credit: (David Pogue)
- Courtesy David Pogue
Participants
- Joanna Aizenberg
- Materials Scientist, Harvard University
- Angela Belcher
- MIT
- Benjamin Charrow
- Julia Herr
- Biologist, University of Guelph
- Vijay Kumar
- University of Pennsylvania
- Atsuko Negishi
- Materials Scientist, University of Guelph
- Robert Playter
- Boston Dynamics
- Marc Raibert
- Founder, Boston Dynamics
Preview | 00:30
Full Program
Notice: Undefined index: video_length in /home/nova/zend/application/templates/resources/multi-ep-pro.phtml on line 500
Full program available for streaming through ...
Watch Online
Full program available
Soon
Making Stuff Colder
Host David Pogue asks if cold holds the key to technology that can improve our lives. Airing October 30, 2013 at 9 pm on PBS Aired October 30, 2013 on PBS
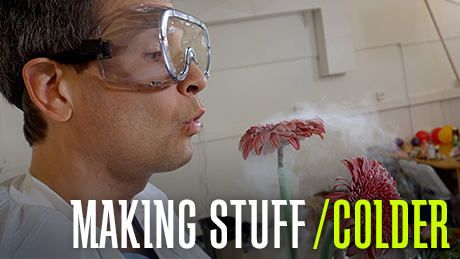
Program Description
Transcript
Making Stuff: Colder
PBS Airdate: October 30, 2013
DAVID POGUE: Cold is the new hot.
Join me on a journey down the thermometer, away from the warm world we inhabit, to the realm of cold.
Cold is a force we can harness to save us, the stuff we are made of.
GREG FAHY (21st Century Medicine): The ability to preserve organs for transplantation.
DAVID POGUE: â¦and even bring us backâ¦
NEWSCASTER: â¦back from the dead.
DAVID POGUE: â¦came back to life.
MARK B. ROTH (Fred Hutchinson Cancer Research Center): Oh, yeah.
DAVID POGUE: We are going to a topsy-turvy world, where heat is the enemy...
ERIC CORNELL (JILA/National Institute of Standards and Technology): Heat is like noise.
DAVID POGUE: â¦random energy and vibration that disturbs and destroys...
ERIC CORNELL: But if you get things really, really cold, you can listen to what nature is whispering to you.
DAVID POGUE: â¦so cold that ordinary physics breaks down and the rules change. Things levitateâ¦
We will travel to a place so cold that new states of matter are born, where cold is creating a new breed of computers, a quantum leap beyond the most powerful today.
GEORDIE ROSE (D-Wave Systems, Inc.): By the time you get to about 500 bits, you have more possibilities than there are atoms in the visible universe.
DAVID POGUE: I'm David Pogue. Join me tonight, as we journey down the thermometerâ¦
MARTIN ZWIERLEIN (Massachusetts Institute of Technology): This is the coldest spot in the universe right now..
DAVID POGUE: That's amazing. I want to get its autograph.
â¦to make stuff colder.I'm beginning my voyage down the thermometer from a very warm place in the universe Earth. Now, you may think it gets pretty cold here. The lowest temperature ever recorded was minus-128 degrees Fahrenheit, in Vostok, Antarctica, in 1983. But that's a heat wave compared to Saturn, Pluto and most of outer space.
We'll get a lot colder than that as we journey down into the weird world of cold, towards absolute zero.
But I'm getting ahead of myself. Our first stop is one we can all relate to: 98.6 degrees, the temperature of my body. Before I came hereâ¦
JOHN CASTELLANI (United States Army Research Institute of Environmental Medicine): We're going to go into the chambers.
DAVID POGUE: The chambers? Sounds ominous.
Turns out, to learn about the cold, first, I have to get hot.
Eighty-two-point-nine? That really doesn't sound so bad.
JOHN CASTELLANI: We are going to put you a little hotter than that today.
DAVID POGUE: John Castellani is a scientist at the Army's Doriot test chamber.
Wow.
JOHN CASTELLANI: Yeah, so this is our environmental chamber: 60 feet long...
DAVID POGUE: This gigantic room is designed to re-create every conceivable environment that a soldier might face, from the frigid mountains of Afghanistan, to the 120-degree deserts of Iraq.
JOHN CASTELLANI: â¦with the wind, and the humidity control.
DAVID POGUE: In order for this place to work, there's one more thing they need: volunteers.
Will we be seeing one of these poor victims.
JOHN CASTELLANI: The only victim that we're going to see in here today is yourself.
DAVID POGUE: Castellani "enlisted" me to help test ways of keeping soldiers cool in the heat. To do this, they'll need to track my vital signs.
MARISSA SPITZ: For core temperature, you'll be using this rectal probe.
DAVID POGUE: Rectal probe?.
MARISSA SPITZ: It may feel a bit uncomfortable, but.
DAVID POGUE: No kidding.
MARISSA SPITZ: â¦all the way past, to make sure it doesn't fall out.
DAVID POGUE: How about I just go in there and tell you how I am? Pretty hot.
MARISSA SPITZ: This is mostly for your safety.
DAVID POGUE: Oh, thanks for thinking of me.
Next, they outfit me in 40 pounds of body armor. And then, it's into the chamber.
JOHN CASTELLANI: It's a hundred and four degrees in here.
DAVID POGUE: A hundred and four.
JOHN CASTELLANI: Yes.
DAVID POGUE: Can I get a little lemon.
Within minutes, my temperature is going up.
What is that? An hour.
WOMAN: Actually, it's been about four minutes.
JOHN CASTELLANI: Their heart rates will start to rise, their core temperatures start to rise.
Your core temperature is around 101.5 degrees Fahrenheit.
Eventually, what will happen is they'll become a heat exhaustion casualty.
DAVID POGUE: After two hours, I called it quits.
Please tell me there was some valid scientific purpose for that.
JOHN CASTELLANI: There is. I mean, really, what we're trying to understand is—when we develop this kind of gear—is can we develop it in such a way that we can allow the body to be able to be able to get rid of the heat?
DAVID POGUE: Oh, man.
And why did I get so hot? Because of some basic laws of physics, which I seem to have missed in my high school science class. For example: What is heat? What is temperature? And what is cold.
EDWARD YARMAK, JR. (Arctic Foundations, Inc.): What did they teach you.
WOMAN: Heat is energy.
ERIC CORNELL: Disorganized energy.
BRIAN WOWK (21st Century Medicine): The vibrations of molecules.
ERIC CORNELL: The motion of atoms.
DAVID POGUE: The faster they move around, the more energy they have. That's heat. Temperature is a measure of how much heat energy they have.
ERIC CORNELL: Temperature is measuring the motion of atoms.
DAVID POGUE: Okay, so heat really is something. It's energy, motion, and you can measure how much of it you have.
ERIC CORNELL: More and more heat, your temperature goes up.
DAVID POGUE: But cold is another story.
ED YARMAK: Because there's no cold flow, there's heat flow.
DAVID POGUE: Really.
ERIC CORNELL: If you take heat out, your temperature goes down.
DAVID POGUE: You mean, you don't put cold in? No.
ED YARMAK: No. No. There's really no such thing as "cold."
DAVID POGUE: So cold is just the absence of heat? So things don't ever really get colder.
ED YARMAK: No, they just get less hotter.
DAVID POGUE: Yeah, that has a great ring to it. "Making Stuff: Less Hotter." That just seems backwards.
JOHN CASTELLANI: So, I have a spoon here for you.
DAVID POGUE: But Castellani showed me that how we think "cold" works.
Cold is just rushing right into my flesh.
â¦can really be the opposite of what's happening.
JOHN CASTELLANI: Actually the heat from your hand is moving into the spoon, not the other way around. Heat moves from areas of hotter temperatures, or high energy, to areas of lower temperature, or low energy.
DAVID POGUE: You can see it through the eyes of this thermal camera, where warm things, like my hand, show up as light orange, and colder things like the spoon are dark.
And sure enough.
JOHN CASTELLANI: If you put the spoon up against your hand, we can see, heat's going from your hand into the spoon.
DAVID POGUE: That's crazy.
The reason I was getting so hot on my forced march was the air was hotter, at a higher energy state, than my skin. So, instead of the heat from my core flowing out to the room, the heat from the room flowed into my core.
The army has a cool solution.
JOHN CASTELLANI: So, David, this is how we're going to cool you off. So what this is is a cooling garment. It's essentially going to circulate water through here. We're going to hook this up to a small refrigerator.
DAVID POGUE: Ooh! I just got chills.
JOHN CASTELLANI: â¦and keep it at about a nice, cool 70 degrees.
DAVID POGUE: I don't know, but I've been told.
SOLDIERS: I don't know, but I've been told.
DAVID POGUE: â¦hundred and four degrees gets old.
SOLDIERS: â¦hundred and four degrees gets old.
DAVID POGUE: Just like last time, my core temperature rises in the 104-degree environment. But this time, the heat's got somewhere to go: into the vest, and out through the refrigeration unit.
JOHN CASTELLANI: A significant difference: 82 degrees Fahrenheit, compared to last time, when it was up around 95 or 96 degrees.
DAVID POGUE: A vest is best if it is cold.
SOLDIERS: A vest is best if it is cold.
JOHN CASTELLANI: He is feeling better. He seems in better spirits, and those are all the benefits that we see with this particular technology.
DAVID POGUE: An hour and a half later, as they remove my Kevlar vest, that dark blue area, that's my chest: 78 degrees. Cool, baby.
But this is just the beginning of what Castellani has in store for me.
Forty-one degrees, that's the temperature in the chamber, as ice water rains down on me.
That's cold.
JOHN CASTELLANI: We're going to do this for 10 minutes.
DAVID POGUE: Ten minutes.
JOHN CASTELLANI: So, to kind of give you an idea of what's going on with you physiologically right now.
DAVID POGUE: I know what's going on with me physiologically! I'm turning into an ice cube.
JOHN CASTELLANI: Yeah.
DAVID POGUE: Castellani and his colleagues developed this procedure, not to torture folks, but to solve a mystery. In 1995, a squad of army rangers waded through a swamp in Florida. Within hours, four died of hypothermia, yet the temperature was 59 degrees.
To understand why they died in such temperate conditions, the army developed this experiment.
So after my cold shower?
A 30-minute march.
JOHN CASTELLANI: We're going to increase the wind.
DAVID POGUE: â¦in 15-mile-an-hour wind.
JOHN CASTELLANI: To mimic, again, you're outside.
DAVID POGUE: He repeats this.
JOHN CASTELLANI: We're going to have it rain on you again.
DAVID POGUE: â¦over and over.
Suddenly, I'm dreaming of the days when it was a hundred and four.
Their investigation discovered that it was this combination of wet, cold and wind that killed the rangers.
JOHN CASTELLANI: So, if you were, say, in 50-degree water and immersed to the chest, that water is conducting so much more heat away from you than would air.
DAVID POGUE: I can see that.
Their research established safety guidelines for troops in the cold.
JOHN CASTELLANI: We may be able to tell people, you know, you may be able to last maybe an hour or an hour and a half, in those kinds of conditions.
DAVID POGUE: What are the early onset signs of hypothermia.
JOHN CASTELLANI: Well, certainly, very intense shivering.
DAVID POGUE: Check.
JOHN CASTELLANI: Changes, for example, in a person's ability to walk or their gait.
DAVID POGUE: What.
JOHN CASTELLANI: They start to grumble, they start to mumble.
DAVID POGUE: [Mumbles]
JOHN CASTELLANI: Three, two, one, go.
DAVID POGUE: The test is designed to go on for six hours, but after just an hour of this, it was time for my career as an army test subject to come to an end.
JOHN CASTELLANI: I think we're going to call it quits now, okay.
DAVID POGUE: So far, I've learned how deadly hypothermia can be, but as I continue down the thermometer to our next stop, 91.4 degrees, I'm about to discover that sometimes the opposite can be true.
CLIFTON CALLAWAY (University of Pittsburgh): This is actually a device to induce hypothermia in patients.
DAVID POGUE: Excuse me! Have you never heard of "First, do no harm?" Hypothermia kills you.
Dr. Clif Callaway is a professor at the University of Pittsburgh.
CLIFTON CALLAWAY: There are situations in which hypothermia can be beneficial, for example, patients after cardiac arrest.
DAVID POGUE: Patients like Susan Koeppen, a mother of three. A few years ago, she set out for a run with friends.
SUSAN KOEPPEN (Cardiac Arrest Survivor): It was a beautiful Sunday morning, in November.
DAVID POGUE: She had no idea what was in store.
SUSAN KOEPPEN: We're about two miles into the run.
DAVID POGUE: When a heart valve suddenly failed.
SUSAN KOEPPEN: I put my hands on my knees, and then collapsed on the sidewalk.
CLIFTON CALLAWAY: Her heart stopped.
SUSAN KOEPPEN: I was gone.
CLIFTON CALLAWAY: Cardiac arrest; paramedics arrived. They were able to stabilize her and transport her to the hospital.
DAVID POGUE: Fortunately, Callaway and his colleagues were there.
Their goal: to stop the brain damage that immediately follows cardiac arrest.
CLIFTON CALLAWAY: She was in a coma. We used cooling blankets for hypothermia therapy.
DAVID POGUE: To prevent permanent brain damage, in the aftermath of cardiac arrest, they dropped her body temperature to 91 degrees. Two days later, they warmed her back up. And soon after,.
CLIFTON CALLAWAY: She came around and talked to her husband for the first time.
SUSAN KOEPPEN: I said, "Where am I?
(Susan Koeppen's Husband): You're in Shadyside Hospital, Honey.
SUSAN KOEPPEN: Why.
(Susan Koeppen's Husband): You never made it home from your run on Sunday.
DAVID POGUE: A year later, she had fully recovered.
SUSAN KOEPPEN: Hypothermia treatment saved my life, saved my brain, and I'm a mom and a wife, like I was before.
DAVID POGUE: The procedure that saved her life is called therapeutic hypothermia, and I'm about to discover how it works.
VOICE ON MACHINE: Therapy started.
DAVID POGUE: I recognize her. She's the lady on the Star Trek: Enterprise.
CLIFTON CALLAWAY: This device has water coming in through this tubing and pulls heat out of your body.
DAVID POGUE: Very cold.
Just like the army's cooling vest, but here, in order to fight brain damage, Callaway brings down body temperatures to 91 degrees.
CLIFTON CALLAWAY: It lowers the metabolism, it reduces brain swelling, it reduces the likelihood of having seizures.
DAVID POGUE: This has proved remarkably effective.
CLIFTON CALLAWAY: The odds of waking up are almost two to three times greater for the patient with hypothermia treatment, compared to the patient without.
DAVID POGUE: But, amazingly, in North America only 40 percent of cardiac arrest patients get this treatment.
Wait. It triples your chance of survival, but only 40 percent of patients get the treatment.
CLIFTON CALLAWAY: Yeah, it's disappointing. We really wish it was done more reliably for more patients.
DAVID POGUE: Yeah, me too.
But there's a limit. His treatment can only save the fortunate few cases where paramedics bring back a heartbeat within minutes. Many trauma patients die on the way to the hospital.
But biochemist Mark Roth says he has a way to save many of them: by getting colder, a lot colder.
MARK ROTH: Well, a simple way to think about it, David, is that we're trying to take the "emergency" out of "emergency medicine.
DAVID POGUE: For years, he's been trying to develop a method that could one day buy trauma patients time, by dropping their core temperatures down as far as 60 degrees. The problem is that when people get that cold, it usually kills them—usually.
MARK ROTH: There are these outliers.
DAVID POGUE: He believes the answer to saving thousands of lives lies within these mysterious cases; cases of people who suffered hypothermia so severe, it stopped their hearts, and yet they came back to life.
Consider the case of Janice Goodger.
NEWSCASTER: â¦to the brink of death and back.
DAVID POGUE: Her heart stopped. She was unconscious, in the freezing snow, for four hours.
MARK ROTH: She was brought to the hospital. Twenty-four hours later, walked out of the hospital, refusing any treatment, and has been fine since.
Her core temperature dropped to 70 degrees.
Another example: Erika Nordby, in Canada.
DAVID POGUE: A one-year-old, her core temperature dropped to 61 degrees, after she wandered into freezing cold weather, wearing nothing but a diaper.
MARK ROTH: They didn't find her 'til morning.
DAVID POGUE: After two hours without a heartbeat, she too was revived.
MARK ROTH: â¦and also made a full recovery.
DAVID POGUE: But no one's gotten colder than Anna Bagenholm.
MARK ROTH: She has the record for the lowest core temperature, at 56.7 degrees Fahrenheit.
NEWSCASTER: She was skiing down a waterfall gully near Narvik, in north Norway, when she fell head first into a river.
BRYANT GUMBEL (News Clip): â¦clinically dead for three hours.
DAVID POGUE: Three hours.
MARK ROTH: Right, right.
NEWSCASTER: It took doctors nine hours to revive her.
DAVID POGUE: She came back to life.
MARK ROTH: Oh, yeah. Oh, yeah.
BRYANT GUMBEL (News Clip): Anna Bagenholm is back at work and well enough to be with us.
DAVID POGUE: In each of these cases, their hearts stopped beating for hours, yet their brains weren't damaged by lack of oxygen.
MARK ROTH: How do the exceptions come to be? There must be some way to exist out there and not require oxygen, there has to be, or these people would be dead.
DAVID POGUE: He is searching for a way to do the same for trauma patients: curb their demand for oxygen and not damage the brain, by cooling them way down.
But there is a big problem.
MARK ROTH: If you're trying to use the cold to create medical benefit, there's a sort of fundamental problem. Mammals fight that, and they make heat, using up resources in your body in order to do that.
DAVID POGUE: As I discovered when I was shivering in the army's test chamber, when people get cold, their metabolism actually increases and they burn more oxygen to make heat.
That's what killed the rangers in the swamp. As they fought to stay warm, their bodies burned through all the available calories, starving their brains of oxygen.
MARK ROTH: Because that's the fuel that, once you burn through it, you are dead.
DAVID POGUE: Roth knew that the reason that Anna Bagenholm and the others survived and the rangers didn't, is that they were able to somehow shut off their body's demand for oxygen. But how could he do the same.
MARK ROTH: So how do I do that? That was a real puzzle.
DAVID POGUE: The answer came to him one night, while he was watching TV.
MARK ROTH: While sitting on my couch at home, watching a NOVA show about a cave in Mexico, they said cave air had a little bit of hydrogen sulfide in it..
SCIENTIST ON NOVA (NOVA Film Clip): So we wear these gas masks to help filter out the hydrogen sulfide.
MARK ROTH: And she said that if you go in there without this respirator, then you will collapse to the ground. Immediately I thought, "That's it!
DAVID POGUE: He thought that hydrogen sulfide might just be the key. He knew that it naturally occurs, in small quantities, in the brain, where it helps the cells regulate oxygen consumption. But he also knew that too much of it overwhelms the cells, turning off their ability to absorb oxygen, starving them.
He wondered if he added just a little to the air, to increase the amount in the brain by just a minute quantity, that instead of starving the brain, he could drastically reduce its need for oxygen.
He tried it on mice.
MARK ROTH: â¦room air laced with hydrogen sulfide.
DAVID POGUE: After three hours, its core temperature drops almost 30 degrees.
MARK ROTH: The mouse is hovering now at about 70 degrees Fahrenheit.
DAVID POGUE: Usually the mouse would fight the cold and burn through its supply of oxygen.
MARK ROTH: The mouse no longer responds to cooling by making heat; it actually just gets colder.
DAVID POGUE: Because of the hydrogen sulfide, its brain's demand for oxygen has dropped by 90 percent.
MARK ROTH: An animal in this state would survive otherwise lethal oxygen deprivation.
DAVID POGUE: Roth thinks that he can do the same for people. The goal is to clinically duplicate the miracle that saved these people's lives by delivering hydrogen sulfide intravenously. And if he can do that, he will revolutionize emergency medicine and save thousands of lives.
It seems the farther down the thermometer we go, the more potential cold has for saving lives. So why stop at 60 degrees? Why not get even colder, to freezing, like in the movies.
Prometheus clip: Suspended animation.
DAVID POGUE: Yes! Suspended animation.
Prometheus clip: It's inevitable.
DAVID POGUE: â¦to make human time capsules.
Austin Powers clip: Powers volunteered to have himself frozen.
DAVID POGUE: â¦or to travel to another solar system.
2001: A Space Odyssey clip: I tucked my crew in for the long sleep.
DAVID POGUE: But there's a reason this is called science "fiction." The human body is about 60 percent water. And when water changes from a liquid into ice, the molecules stop moving around freely and lock together to form crystals, and that destroys cells.
So far, no one has been able to get around that problem with people, but there is a creature that has.
JON COSTANZO (Miami University): So we find it, generally, under the leaf litter.
DAVID POGUE: Cryobiologist Jon Costanzo studies an animal that has beaten the problem of freezing.
Hey.
â¦the North American wood frog.
JON COSTANZO: Some of these animals can, in fact, survive freezing and thawing of their body fluids.
DAVID POGUE: Back at his lab, he pulled one out from a deep freeze.
JON COSTANZO: Let's go take a look.
DAVID POGUE: Whoa. Oh, man.
JON COSTANZO: There's no heartbeat. There's no brainwaves..
DAVID POGUE: A dead frog.
JON COSTANZO: No. No, it isn't.
DAVID POGUE: It's a brick of ice.
JON COSTANZO: It's very much alive.
DAVID POGUE: If there's no brain activity, it's dead.
JON COSTANZO: Clinically, perhaps, but we've seen them thaw and come back to life.
DAVID POGUE: How would that be possible? Ice destroys cells, right.
JON COSTANZO: This frog has worked out a number of different ways to avoid that kind of damage.
DAVID POGUE: The frog's vital organs shrivel up, releasing their water safely away from the frog's organs. And something else happens.
JON COSTANZO: Most importantly, as soon as the frog begins freezing, the liver begins producing compounds that allow the cells and tissues to survive.
DAVID POGUE: A kind of antifreeze, or as Costanzo calls them.
JON COSTANZO: Cryoprotectants.
DAVID POGUE: Cryoâ¦
JON COSTANZO: â¦protectants, in huge quantities.
DAVID POGUE: Which protect the frog until the spring, when something amazing happens.
JON COSTANZO: The ice begins to melt, and water returns to its usual location. So, the cells take the water back up. And, after a time, the heart begins beating again. We don't know how this happens; it just spontaneously resumes beating.
DAVID POGUE: That's crazy.
JON COSTANZO: That's one of the first signs that the frog is really not dead at all. It's alive. And then the frog begins to breathe. Eventually the frog will be able to move its limbs, sit upright, and, eventually, it can hop away.
DAVID POGUE: With these cryoprotectants, the frog has survived the cold of winter.
If we could figure out a way to do this for people, we could save lives. Not by freezing our bodies, but by preserving our organs for transplantation. That's because organs, even on ice, have a limited shelf-life. Hearts, for example, last, at most, six hours.
Thousands of people die each year waiting for an organ.
So, could we not just inject these cryoprotectants into our bodies.
JON COSTANZO: Unfortunately, it doesn't work that way. Some of the cryoprotectants that these frogs use are very toxic to mammalian muscle tissue.
DAVID POGUE: So, how to come up with a similar chemical for human organs? That's the problem researcher Greg Fahy is trying to solve.
GREG FAHY: The ultimate goal is to be able to set up real banks of organs, so that they can be moved anywhere, ready to be plugged in within a couple of hours' notice.
DAVID POGUE: After years of work, he thinks he may have come up with a cryoprotectant that's safe for mammals.
GREG FAHY: Unlike the chemicals that the frog uses, we have optimized this particular mixture for the mammal, over the last 30 years or so.
DAVID POGUE: With this mixture, Fahy has successfully preserved rabbit kidneys at below freezing temperatures.
He starts by removing as much water as possible.
GREG FAHY: So, the kidney might start off being 80 percent water. We're going to reduce it to about 30 percent water.
DAVID POGUE: All right, so water out, antifreeze in.
GREG FAHY: Yes.
DAVID POGUE: The "antifreeze" is the key. It's called M22, a strange substance that's not toxic to rabbits or humans.
BRIAN WOWK: And, as you can see, at room temperature, it's clearly a liquid.
DAVID POGUE: I imagine M22 must, of course, work better than M20 and M21 did.
GREG FAHY: Well, M22 is named because it's intended to be used at minus-22 degrees Celsius, which is minus-eight degrees Fahrenheit.
DAVID POGUE: And when they take it down below those temperatures, it behaves strangely.
BRIAN WOWK: Cooled to below minus-100 degrees Fahrenheit.
DAVID POGUE: Whoa.
BRIAN WOWK: â¦and it's now like a viscous syrup.
DAVID POGUE: Pump this into a kidney, and no matter how cold the organ gets, it won't freeze.
How does that work.
Unlike solid ice, where molecules are tightly organized, Fahy's cryoprotectant remains liquid, no matter how cold it gets.
GREG FAHY: At a certain point, there's insufficient heat energy in the system to maintain molecular motions, and the system just locks up as a solid. But it's not a frozen solid. It's going to a very different kind of solid state.
DAVID POGUE: So it's a solid, but it's not ice.
GREG FAHY: It's called a glassy solid state, sort of like a windowpane.
DAVID POGUE: So it's not called "freezing" organs, you'reâ¦
GREG FAHY: Vitrifying those organs.
DAVID POGUE: Vitrifying.
It took them about four hours to bring the kidney into this state.
BRIAN WOWK: This is now solid. The kidney, and the solution surrounding it, is at a temperature of minus-190 Fahrenheit. It is a solid glass through and through.
DAVID POGUE: Frozen only in time.
GREG FAHY: It's just like it was in the liquid state. The only difference is that nothing in the liquid can move anymore. And, of course, if nothing can move, nothing can change. If nothing can change, then you have perpetual preservation.
DAVID POGUE: Forever.
GREG FAHY: Well, practically.
DAVID POGUE: A hundred years?
GREG FAHY: Forever, as far as you're concerned.
DAVID POGUE: Fahy and his team have successfully re-implanted one of these kidneys into a rabbit.
GREG FAHY: And we believe that we can put any organ into a vitrified state with enough effort and time.
DAVID POGUE: By making organs colder, without actually freezing them, he hopes to make organ-banking a reality.
GREG FAHY: If we can do that, then that organ can wait as long as it takes for the right person to come along who needs it.
DAVID POGUE: From cooling soldiers and saving heart attack victims, to preserving organs, the cold has amazing potential. And, as I continue my journey down the thermometer, moving from the world inside us to the world around us, I'm headed to the last place you'd think anyone would want to make colder: Fairbanks, Alaska. I trekked there to find out why.
Life here seems to revolve around snow and ice, whether it's just play or beautiful works of fine art. It's very handsome.
And while snow blankets the ground from early fall to late spring, much of the earth underneath stays frozen year round. It's called permafrost, but there's a big problem: it's not so perma. When you put a heated building on it, or even an asphalt road, permafrost melts.
It wasn't always wavy like this.
DOUG GOERING (University of Alaska Fairbanks): Oh, no. When this road was first constructed, it was perfectly level.
DAVID POGUE: Houses are sinking into the ground.
DOUG GOERING: You can see that many of them are not particularly level.
DAVID POGUE: And this doesn't just happen overnight.
WOMAN: No, of course not. It started about 40 years ago, maybe 30-something. No one ever thought it would get like that.
DAVID POGUE: You must've noticed that things were tipping a little bit. Your coffee cup would slide across the table.
WOMAN: Well, it wasn't that extreme, 'cause anyone with common sense would level the table, no matter what condition you're in.
DAVID POGUE: Good point.
But what exactly is permafrost anyway.
DAN WHITE: Watch your head.
DAVID POGUE: Dan White, of the University of Alaska Fairbanks...
Whoa.
...took me underground to find out.
Welcome to my lair, Mr. Bond.
This tunnel is the Army and University of Alaska's permafrost joint research facility.
DAN WHITE: Everything here is permafrost.
DAVID POGUE: When it comes to building houses and roads, there are two different kinds of permafrost.
DAN WHITE: Gravelly materials.
DAVID POGUE: â¦the kind you can build on.
DAN WHITE: If you had a building, a road, on top of this, and you thaw that out, it would remain stable.
DAVID POGUE: The other kind of permafrost is the problem.
DAN WHITE: Fine grain soil.
DAVID POGUE: â¦which gets its structural integrity from frozen water that acts as cement.
DOUG GOERING: So long as it's in the frozen state, you can see that it's structurally sound. You can build roads or bridges or houses on something like this.
DAVID POGUE: Mm hmm.
DOUG GOERING: The problem, though, is that once it warms up, it turns into this.
DAVID POGUE: A scientific principle we call "melting..
DOUG GOERING: Exactly.
DAVID POGUE: And it's only going to get worse as global temperatures rise. So how to stop it?
ED YARMAK: We use these thermosiphon devices.
DAVID POGUE: Thermosiphons. Ed Yarmak is chief engineer with the company that invented these things.
ED YARMAK: Well, it's pretty simple; it's just a tube.
DAVID POGUE: Here's how it works: first, you put some liquid in. Next, you suck out all the air to create a vacuum. Then you get something cold.
ED YARMAK: I've got a little Fairbanks snow.
DAVID POGUE: Remember how things always move from hot to cold? Well, because the snow is colder than the air in the roomâ¦
Whoa! It's going nuts!
â¦the cold draws the heat from the room into the tube, and.
It's cool to the touch, it can't be boiling.
Why is it doing that.
ED YARMAK: Your boiling point is dependent, not only on temperature, but on the pressure inside your tube or in your system.
DAVID POGUE: Because it's in a vacuum, it boils at room temperature, moving the heat from the room into the snow.
Okay, got it. But how in the world is this going to save the permafrost.
Well, when you place one of these in permafrost, the heat from the permafrost moves into the thermosiphon.
One doesn't think of permafrost as having heat.
ED YARMAK: Everything has heat, David. In the wintertime, the permafrost is warmer than the air above it. And we all know that heat goes from warm to cold.
DAVID POGUE: Okay, so the heat from the permafrost moves into the thermosiphon. The liquid inside boils, turning into a gas, which rises up, carrying the heat with it. When it gets to the surface, the heat moves out into the colder air.
So it stops thawing.
ED YARMAK: Exactly.
DAVID POGUE: And that works.
It's true. In Fairbanks, you can see them around buildings, in roads, and along the 800 miles of the Alaskan pipeline, you'll find 124,000 of them.
Okay, so right now, the building's heat would be thawing the permafrost, except that these devices are sucking the heat out, right.
ED YARMAK: Exactly.
DAVID POGUE: â¦blasting it into the colder air? But I've found a problem with your system. In the summer, the air out here is not cold, so it would not be sucking heat out. I've got you.
ED YARMAK: Well, in the wintertime, we supercool it, so to speak.
DAVID POGUE: So that it will have excess cold for the summer.
ED YARMAK: Exactly. All summer.
DAVID POGUE: Okay, well what evidence do I have that it's actually working?.
ED YARMAK: You can use this thermal-imaging camera.
DAVID POGUE: Oh, wow. They're glowing.
You remember the thermal camera. It sees cold areas as darker and warmer areas as lighter.
So, we can see that there is heat coming out of them there pipes, but there's only one way to know for sure.
Ahh, ahh, ahh! I'm just kidding..
But Fairbanks is hot compared to where we're going. We're plummeting over 200 degrees colder than any place on Earth, where physicists say a whole new world begins: minus-320 degrees Fahrenheit. That's the temperature of this liquid nitrogen.
And so much fun! Liquid nitrogen, folks, the ultimate in cold.
ERIC CORNELL: Actually, nowhere near the ultimate in cold.
DAVID POGUE: Physicist Eric Cornell knows cold.
ERIC CORNELL: Liquid nitrogen isn't even close.
DAVID POGUE: He and his colleagues won a Nobel Prize for using it to discover a new state of matter. Cornell says we're headed to a place so cold that someone had to invent a whole new thermometer just to get there.
ERIC CORNELL: Yes, Kelvin, an entirely different one, where zero really means something: the bottom, the very lowest temperature you can get to. We call it "absolute zero."
DAVID POGUE: But this, this has a zero on it.
ERIC CORNELL: Yeah.
DAVID POGUE: So, check this out. Check this out.
ERIC CORNELL: It went down to zero. And look, I've got an amplifier that goes up to 11. It doesn't mean anything.
DAVID POGUE: The "Kelvin," in the Kelvin scale, is 19th century physicist Lord Kelvin, who wondered, if temperature is a measure of atomic motion, with less and less the farther down you go, why not make zero the place where all motion would stop?
He calculated that would happen at minus-459-point-six-seven degrees Fahrenheit, which he made zero on his scale.
ERIC CORNELL: A real zero, an absolute zero.
DAVID POGUE: So, in the Kelvin world, room temperature would beâ¦
ERIC CORNELL: About 300.
DAVID POGUE: And water freezes atâ¦
ERIC CORNELL: Two-hundred-seventy-three Kelvin.
DAVID POGUE: And this liquid nitrogen.
ERIC CORNELL: Seventy-seven Kelvin. Truth of the matter is, compared to where we're going, 77 Kelvin is positively balmy. In fact, as you get things colder and colder, that's actually when they start to get the most interesting.
DAVID POGUE: Indeed.
To continue on, we'll need to shed our Fahrenheit scale, and replace it with Lord Kelvin's. Then take a rapid plunge, from 77 degrees Kelvin down to.
MELISSA GOOCH (University of Houston): Four Kelvin.
DAVID POGUE: â¦four Kelvin, where the bizarre property of superconductivity was first discovered.
MELISSA GOOCH: There are certain materials that when you get it really cold, weird things happen.
DAVID POGUE: Little did I know that the substance that would take us there really isn't bizarre at all.
MELISSA GOOCH: Helium.
DAVID POGUE: It's really not that cold; that's the funny thing.
MELISSA GOOCH: But if we turn it into liquid, it's four Kelvin, so, really cold.
DAVID POGUE: Melissa Gooch, at the University of Houston, is about to show me how when certain materials.
This is a piece of lead.
MELISSA GOOCH: This is lead.
DAVID POGUE: â¦get supercold, they start behaving in ways once thought impossible. To do this, she lowers the lead into this tank of liquid helium, to cool it to a temperature of seven degrees Kelvin.
It looks like the temperature is plummeting into warp core, Captain.
MELISSA GOOCH: If we keep cooling.
DAVID POGUE: That ordinary lump of lead, in there, undergoes a transformation.
Is it, in fact, a superconductor.
MELISSA GOOCH: Yes.
DAVID POGUE: Wait. What is a superconductor? Or for that matter, what's a regular conductor.
Conductors are materials that allow electricity to flow through, like copper. Most of the wiring in your house is copper. But copper has a problem, when electricity flows through it, electrons bump around, wasting energy as heat. That's called resistance. A superconductor has no resistance, zero. So the current flows through it without wasting any energy.
MELISSA GOOCH: This is copper wire that we have in our house, normally.
DAVID POGUE: And these are normal light bulbs. But Gooch is going to run much less power through them than normal.
MELISSA GOOCH: So we're at 12 volts.
DAVID POGUE: That's only a tenth of the voltage we use in our homes.
MELISSA GOOCH: It's not very bright.
DAVID POGUE: That's what we get from the copper wire. Watch what happens when we run the same 12 volts through the superconductor.
From a dull glow, to full throttle. So you're getting a lot more out of your electricity.
MELISSA GOOCH: You're getting a lot more out of it.
DAVID POGUE: Oh. You're wasting a lot less.
MELISSA GOOCH: Yes.
DAVID POGUE: Scaled up, that wasted energy, just in the United States grid alone, could power 14 New York Cities every year. But that's just the start. Scientists have been working on harnessing the properties of superconductivity for much more exotic applications.
ALAIN SACUTO (Université Paris Diderot, Paris 7): Now, I put the superconductor.
DAVID POGUE: Here at the University of Paris, Professor Alain Sacuto showed me something extraordinary that happens when a really cold superconductor meets an ordinary magnet.
ALAIN SACUTO: And there is levitation, you know.
DAVID POGUE: And this little puck is just the tip of the iceberg.
Whoa, come on.
ALAIN SACUTO: Let's go.
DAVID POGUE: Back to the future! I'm actually surfing above the ground. I'm flying.
And it's more than just fun and games. Engineers in Japan are already scaling it up to create the world's first superconductor Maglev passenger train. It flies above its tracks at speeds up to 311 miles per hour. And it's cold that makes it happen. So what's the trick.
You might think that the superconductor is acting just like a magnet, but you'd be wrong.
ALAIN SACUTO: It's not like a magnet, because here you have both repulsion and attraction.
DAVID POGUE: So these two discs have repulsion and attraction?
ALAIN SACUTO: Both.
DAVID POGUE: And that's not how a proper magnet behaves. It can't do both at the same time. The superconductor can, because it warps the magnetic field of the magnet to a point where it attracts and repels at the same time.
But it's both directions. It's locked.
ALAIN SACUTO: Yes, both directions.
DAVID POGUE: But how is this possible? How do superconductors actually work.
Look at that.
The crazy part is scientists don't really know. It has something to do with that q-word and, okay, where we're going now: two degrees Kelvin. Physicists are unlocking a whole new world of cold, where the laws of nature appear to break down.
You again.
ERIC CORNELL: Yes. Welcome to my world.
DAVID POGUE: What is this place?.
ERIC CORNELL: This is the "matter menagerie," you know? Like in states of matter.
DAVID POGUE: I do know states of matter: solids, liquids, gases.
ERIC CORNELL: Nah. Way more. You've come to, like, a whole zoo of different states of matter that is called "strange matter."
DAVID POGUE: Well named.
ERIC CORNELL: We think that it only exists in the centers of neutron stars. This one, I'm particularly proud of. That is the "Bose-Einstein condensate," and when we discovered that stuff, I won a Nobel Prize.
DAVID POGUE: Nice. I like how you worked that in.
ERIC CORNELL: Yeah, well, you know.
DAVID POGUE: How many more states of matter are there.
ERIC CORNELL: Truth is, some people say hundreds..
Look at that. It's called a "superfluid."
DAVID POGUE: Superfluid?
ERIC CORNELL: Yes. Give that thing a swirl. What do you think is going to happen?
DAVID POGUE: When I stir it? It's going to go around and around in the bucket.
ERIC CORNELL: Give it a shot, a little faster.
DAVID POGUE: A superfluid is a state of matter found at temperatures below two Kelvin.
Oh, weird..
ERIC CORNELL: Now, go a little faster yet. Yeah.
DAVID POGUE: Weird!
And once these get started, they'll swirl forever.
Whoa!
ERIC CORNELL: That is quantum mechanics in action.
DAVID POGUE: As I'm discovering, quantum mechanics is a kind of physics where the usual rules don't apply.
ERIC CORNELL: Think of it this way: in the ordinary world, you, me, atoms, anything you want, they kind of act like balls, just like these balls, here. They bounce, they roll around, they bash off each other. But in the quantum mechanical world, each of the atoms starts to act more and more like a wave. And eventually, the wave of one atom starts to grow into the wave of the other, and before you know it, you can't tell one from the other. The atom could be over here, or it could be over there. And the cool thing is that it could, in some sense, be, really, both at the same time.
DAVID POGUE: Both at the same time?
ERIC CORNELL: Yeah.
DAVID POGUE: I mean, how can something be in two places at once?.
ERIC CORNELL: It's not something we understand that well either, we just go with it.
DAVID POGUE: No kidding!
ERIC CORNELL: Quantum mechanics is like that, yeah.
DAVID POGUE: And so I, we, depart for colder places, to see how this quantum weirdness can be harnessed to solve real-world problems.
To do that we'll need to inch closer, a tenth of a degree, a hundredth of a degree above absolute zero.
GEORDIE ROSE: Welcome to one of the coldest places in the universe.
DAVID POGUE: What? In an office park, in a Vancouver suburb.
Meet physicist Geordie Rose, of D-Wave Systems, who claims they've used the cold to build the world's first commercial quantum computer.
Oh, my gosh! It's C-3PO's wedding cake!
GEORDIE ROSE: This is a quantum computer.
DAVID POGUE: And what does that mean?
GEORDIE ROSE: You have to rethink the way that you think about computers to wrap your head around it.
DAVID POGUE: Remember that quantum strangeness below two Kelvin? Well, inside this giant refrigerator, Rose's team keeps a few atoms a hundred times colder, all to harness those weird abilities to make a new kind of computer.
So, let me get this straight. This entire company, this entire building, this entire meat locker, this entire million-dollar apparatus is all designed just to make that tiny chip cold.
GEORDIE ROSE: Yes.
DAVID POGUE: What does the cold have to do with the computing.
GEORDIE ROSE: In quantum mechanics, the properties that we're trying to harness are very easily washed out by the movement of the atoms in the processor.
As you go down the plates through four Kelvin, point seven, point one. At each stage, we want to remove the wiggling of the atoms so they just calm down, take a seat on the couch, relax. And when they do that, these wonderful, powerful, magical properties that exist in quantum mechanics blossom out.
DAVID POGUE: Quantum properties like being in two places at once: that magical ability allows D-Wave to program their computer in a very special way.
GEORDIE ROSE: The fundamental piece of information storage in this is a device called a qubit.
DAVID POGUE: Like the biblical measurement.
GEORDIE ROSE: Very unlike the biblical measurement.
DAVID POGUE: A qubit is the quantum version of a bit, the basic unit of information. In a regular computer, a bit can be either a zero or a one. But a quantum bit can be either a zero or a one, or both zero and one at the same time. This gives it exponentially more power than a conventional computer, which would use eight bits just to store a single number between zero and 256. In a quantum computer, eight qubits can store all 256 numbers at once.
GEORDIE ROSE: The real kicker is when you have a lot of these bits together, the total number of possibilities doubles every time you add a bit.
DAVID POGUE: So, while ten qubits can store (210) = 1,024 numbers, 11 qubits can store (211) = 2,048 numbers. When you get to 100 qubits, you can store (2100) = 1 nonillion, 267 octillion, 650 septillion, 600 sextillion, 228 quintillion, 229 quartrillion, 401 trillion, 496 billion, 703 million, 205 thousand and 376) number.
GEORDIE ROSE: So by the time you get to about 500 bits, you have more possibilities than there are atoms in the visible universe.
DAVID POGUE: Wow.
What this means is that a quantum computer can tackle problems on a scale beyond any conventional computer: from weather prediction and air traffic control, to forensics and finance. Problems on this scale are everywhere and have simply outstripped our abilities to solve them.
And though some have questioned their claims, there are buyers. D-Wave's first customer? Aerospace giant Lockheed Martin.
Their F-35 fighter plane is incredibly sophisticated.
. And we have touchdown! Whoa! This is a fairly software-dependent little plane.MICHAEL: Yes. It's got about 9,000,000 lines of code.
DAVID POGUE: Nine-million lines of code? Those nine-million lines of code can land a plane on a carrier, evade enemy radar and hover like a helicopter. Trouble is, no conventional computer could ever check that software for errors without an army of engineers.
Is it just too many variables to all consider at the same time?
BRAD PIETRAS (Lockheed Martin): That's exactly right. If you have a million, suddenly you can't manage it with any computer on Earth.
DAVID POGUE: Which is why now NASA and Google partnered to get a quantum computer, too, in hopes of better finding habitable planets and speeding up the search.
Can I have one.
GEORDIE ROSE: How much money you got.
DAVID POGUE: Not enough.
So, D-Wave's chip is one of the coldest things in the universe: a hundredth of a degree above absolute zero.
Well, we're not done yet.
I'm about to meet a scientist who can't be bothered with hundredths or even thousandths of a degree for that matter, a.k.a. a millikelvin.
MARTIN ZWIERLEIN: We are bored by a millikelvin; we like to go to nanokelvin.
DAVID POGUE: Nanokelvin.
MARTIN ZWIERLEIN: Nanokelvin; that would be a billionth of a degree above absolute zero.
DAVID POGUE: A billionth of a degree.
MARTIN ZWIERLEIN: It's very cold! It's a million times colder than interstellar space.
DAVID POGUE: It's just about the lowest temperature ever reached: a place so clear and cold, physicists can see the fundamental laws of nature in action.
M.I.T.'s Martin Zwierlein is going to use sodium atoms to show me how to get there: the final frontier of cold.
Wow. And, so, how do you do that.
MARTIN ZWIERLEIN: So, we can start over there, at the oven.
DAVID POGUE: The oven.
Step 1: Cook up some sodium atoms, the same kind in your table salt, to about 700 degrees Fahrenheit. That way you can separate them.
MARTIN ZWIERLEIN: You want to get single atoms to play with, single sodium atoms, lots of them, a whole stream of them.
DAVID POGUE: Step 2: Hit them with lasers.
I know you M.I.T. guys have the reputation of being very smart, but I have a little tip for you: lasers are hot. You might be a little backwards there.
MARTIN ZWIERLEIN: Yeah. You might think about Star Trek, where they kill people with lasers. Turns out here, we cool atoms down with lasers. They get a recoil from it, just as if you hit a billiard ball with another billiard ball.
DAVID POGUE: In other words, when you hit atoms with just the right amount of laser light, it acts like a little shove in the opposite direction that the atom is moving, slowing it down.
MARTIN ZWIERLEIN: If you look down here, you will actually see the cold cloud, right there in the center of the vacuum chamber.
DAVID POGUE: That glowing star thing? It looks like the sun, it ought to be super, super hot.
MARTIN ZWIERLEIN: No. It's actually extremely cold. Those are a billion atoms, cooled to a millikelvin.
DAVID POGUE: A thousandth of a degree above absolute zero. But lasers can only get us so far.
MARTIN ZWIERLEIN: You cannot reach the nanokelvin temperatures just with laser cooling, so we need another technique.
DAVID POGUE: Which brings us to Step 3: Get out your coffee cup.
MARTIN ZWIERLEIN: What takes over after laser cooling is what we call evaporative cooling. It's the same thing that happens to your coffee right now, because it's just cooling down. So, if you now force it a little bit, by blowing on the coffee, huh? You speed that process up. The coffee gets cold more quickly, that's exactly what we do here.
DAVID POGUE: But instead of a coffee cup, Zwierlein uses a cup made of magnetic fields to trap his atoms. Then he "blows" on it with radiation and lowers the rim of the cup to let the hotter atoms escape.
MARTIN ZWIERLEIN: So, now, we're going to do this coffee-cup cooling. It's going to bring us to nanokelvin, okay?
Ready for this.
DAVID POGUE: Yes.
MARTIN ZWIERLEIN: Let's do this.
So, can you please switch on this stuff? Do this, this is great. Let's switch on this guy, and then this awesome knob, here.
DAVID POGUE: Press the awesome white button.
MARTIN ZWIERLEIN: Fantastic! So, that's good. Please press F12.
DAVID POGUE: I've always wondered what F12 does.
MARTIN ZWIERLEIN: You see, now, that the atoms are cooling, because the cloud size gets smaller and smaller and smaller. Here you see the temperature drop, drop, drop, drop, drop.
DAVID POGUE: Oh, wow.
It takes a few minutes, but eventually the atoms become so cold, they lose their individual identities altogether and coalesce into that new state of matter called a Bose-Einstein condensate.
MARTIN ZWIERLEIN: And that has formed right now.
DAVID POGUE: Wait a minute, wait a minute. Okay.
MARTIN ZWIERLEIN: Yes.
DAVID POGUE: So, that's the condensate.
MARTIN ZWIERLEIN: That's the condensate.
DAVID POGUE: But look at the temperature.
MARTIN ZWIERLEIN: Yeah, it's very cold.
DAVID POGUE: A hundred-and-seventy-seven-billionths of a degree? Billionths.
MARTIN ZWIERLEIN: Billionths, billionths of a degree.
DAVID POGUE: A hundred-and-seventy-seven-billionths of a degree Kelvin.
MARTIN ZWIERLEIN: This is the coldest spot in the universe right now, right here.
DAVID POGUE: That's amazing.
MARTIN ZWIERLEIN: Yep.
DAVID POGUE: So, not even outer space.
MARTIN ZWIERLEIN: No, no, no. Outer space is a million times hotter.
DAVID POGUE: Not the dark side of the moon.
MARTIN ZWIERLEIN: No, it's, like, all hot.
DAVID POGUE: Comets.
MARTIN ZWIERLEIN: Terrible..
DAVID POGUE: Black holes? Nothing.
MARTIN ZWIERLEIN: Nothing.
DAVID POGUE: This is it, in this room. That's amazing. I would ask it for its autograph if I could.
But it's not about setting obscure records. What Zwierlein is excited about is what these exotic states of matter can teach us about the universe.
MARTIN ZWIERLEIN: Our puff of gas teaches us about the neutron stars, or the split second after the Big Bang, there was this weird form of matter called the quark-gluon plasma.
DAVID POGUE: A superhot type of matter in the early universe that would give rise to everything we see today.
So, you're telling me that this tiny, freezing cold dot can teach us something about enormous, blazing-hot stuff?.
MARTIN ZWIERLEIN: That's the fun part of physics. It connects these very different areas. The very hot, very cold, everything is governed by the same laws..
DAVID POGUE: Amazingly, what happens at these ultracold temperatures is that atoms get so smeared out, their waves start looking indistinguishable from those of superhot particles under extreme pressure, like those inside the inner core of neutron stars, so dense, a teaspoon of them weighs 10-billion tons.
Zwierlein and others can now simulate substances like this in their labs and probe their mysteries.
That's incredible. And in a couple more years, you'll finally do it. You'll hit 0.0, absolute zero, and we'll be done.
MARTIN ZWIERLEIN: Yeah, unfortunately, it's never possible to reach absolute zero.
DAVID POGUE: What.
MARTIN ZWIERLEIN: There's always going to be a little, little drop of energy sitting around somewhere.
DAVID POGUE: Turns out, it's impossible to get to absolute zero, because no matter how cold you get, everything has tiny quantum jitters. And where you have motion, even a tiny amount, you have heat.
But that's not stopping scientists from getting even colder to explore the fundamental laws of nature and how our universe came to be.
ERIC CORNELL: Just the way noise can drown out music, heat is sort of the noise that obscures things. If you get things really, really cold you sort of drown out, you damp down all the noise and you can listen to what nature is whispering to you..
DAVID POGUE: It's uncharted territory. Like other frontiers of science, cold has opened the doors to new worlds. Where the dead may get a second chance, the planet can be cooled by clever innovation and the universe may be made more understandable. The secrets are all around us, as we learn to make stuff colder.
Broadcast Credits
- Host
- David Pogue
- WRITTEN BY
- Vincent Liota
- PRODUCED AND DIRECTED BY
- Michael Bicks
Vincent Liota
Anna Lee Strachan - EXECUTIVE PRODUCER
- Chris Schmidt
- ASSOCIATE PRODUCER
- Karinna Sjo-Gaber
- EDITED BY
- Daniel Gaucher
Vincent Liota
Doug Quade - DIRECTOR OF PHOTOGRAPHY
- Jason Longo
- ADDITIONAL CAMERA
- Thomas Danielczik
Stephen McCarthy
Max Miller
Carl Otto - SOUND RECORDISTS
- Fred Burnham
Chad Djubek
Denis Guilheim
Scott Jones
Ken King
Paul Lawrence
Anthony Mariotto
Robert Marts
Jason Pawlak
Paul Rusnak
James Willis
Peter Wong - MUSIC
- Christopher Rife
- ART DIRECTOR
- Ekin Akalin
- ANIMATION
- Vincent Liota
Smash5 Studios
Doug Quade - ADDITIONAL EDITING
- Max Powers
- ONLINE EDITOR AND COLORIST
- Michael H. Amundson
- AUDIO MIX
- Heart Punch Studio, Inc.
- RESEARCH
- Shraddha Chakradhar
Barbara Moran - PRODUCTION ENGINEER
- Karl Siebrecht
- LIGHTING DIRECTOR
- Laszlo Varga
- GAFFER
- Jon Wignall
- PRODUCTION ASSISTANTS
- Ken Longoria
Devin McCloskey
Caleb Souder - ARCHIVAL MATERIAL
- ABCNews VideoSource
AP
Corbis
D-Wave Systems
FleetMon
Getty
iStock
KDKA-TV
Susan Koeppen
Lockheed Martin Corporation
Mayo Clinic
NASA
NASA/Goddard Space Flight Center
NASA/Goddard Space Flight Center, the Advanced Visualization Laboratory at the National Center for Supercomputing
Applications, A. Boley, A. Kritsuk and M. Norman
NASA/Goddard Space Flight Center Conceptual Image Lab
NASA/Goddard Space Flight Center/SDO
Natick Soldier RDEC
Roth Lab, Fred Hutchinson Cancer Research Center
Shutterstock
T3Media
Animations from "Absolute Zero," Windfall Films/Meridian Productions
UPMC
Mira Zimet - Special Thanks to the United States Department of Defense
- SPECIAL THANKS
- Qi Feng An
FLIR Systems
Ice Alaska
Specialist Marissa Spitz
Kristen Heavens - ADVISORS
- Jim Collins
Tony DeRose
Dale Dougherty
Alex King
Tim McGee
Amy Moll
Mike Petrich
Ainissa Ramirez
Thea Sahr
Eric Siegel
Stephen Streiffer
Desney Tan
Jessika Trancik
Thomas Tretter
Phil Weilerstein - NOVA SERIES GRAPHICS
- yU + co.
- NOVA THEME MUSIC
- Walter Werzowa
John Luker
Musikvergnuegen, Inc. - ADDITIONAL NOVA THEME MUSIC
- Ray Loring
Rob Morsberger - CLOSED CAPTIONING
- The Caption Center
- POST PRODUCTION ONLINE EDITOR
- Jim Ferguson
- PUBLICITY
- Eileen Campion
Victoria Louie - SENIOR RESEARCHER
- Kate Becker
- NOVA ADMINISTRATOR
- Kristen Sommerhalter
- PRODUCTION COORDINATOR
- Linda Callahan
- PARALEGAL
- Sarah Erlandson
- TALENT RELATIONS
- Scott Kardel, Esq.
- Janice Flood
- LEGAL COUNSEL
- Susan Rosen
- DIRECTOR OF EDUCATION
- Rachel Connolly
- DIGITAL MANAGING PRODUCER
- Kristine Allington
- SENIOR DIGITAL EDITOR
- Tim De Chant
- DIRECTOR OF NEW MEDIA
- Lauren Aguirre
- DEVELOPMENT ASSOCIATE
- Lisa Leombruni
- UNIT MANAGER
- Ariam McCrary
- PRODUCTION MANAGER
- Stephanie Mills
- POST PRODUCTION ASSISTANT
- Brittany Flynn
- POST PRODUCTION EDITOR
- Rebecca Nieto
- POST PRODUCTION MANAGER
- Nathan Gunner
- COMPLIANCE MANAGER
- Linzy Emery
- BUSINESS MANAGER
- Elizabeth Benjes
- DEVELOPMENT PRODUCER
- David Condon
- PROJECT DIRECTOR
- Pamela Rosenstein
- COORDINATING PRODUCER
- Laurie Cahalane
- SENIOR SCIENCE EDITOR
- Evan Hadingham
- SENIOR PRODUCER
- Julia Cort
- SENIOR SERIES PRODUCER
- Melanie Wallace
- MANAGING DIRECTOR
- Alan Ritsko
- SENIOR EXECUTIVE PRODUCER
- Paula S. Apsell
A NOVA Production by Little Bay Pictures, LLC for WGBH/Boston
© 2013 WGBH Educational Foundation
All rights reserved
This program was produced by WGBH, which is solely responsible for its content.
Image:
- Image credit: (David Pogue)
- Courtesy David Pogue
Participants
- Clifton Callaway
- University of Pittsburgh
- John Castellani
- U.S. Army Research Institute of Environmental Medicine
- Eric Cornell
- JILA/NIST
- Jon Costanzo
- Miami University
- Greg Fahy
- 21st Century Medicine
- Melissa Gooch
- University of Houston
- Susan Koeppen
- Cardiac Arrest Survivor
- Brad Pietras
- Lockheed Martin
- Geordie Rose
- D-Wave
- Mark Roth
- Fred Hutchinson Cancer Research Center
- Alain Sacuto
- Université Paris Diderot, Paris 7
- Daniel White
- University of Alaska Fairbanks
- Edward Yarmak, Jr.
- Arctic Foundations, Inc.
- Martin Zwierlein
- MIT
Preview | 00:30
Full Program
Notice: Undefined index: video_length in /home/nova/zend/application/templates/resources/multi-ep-pro.phtml on line 500
Full program available for streaming through ...
Watch Online
Full program available
Soon
Making Stuff Safer
Host David Pogue examines groundbreaking research that aims to keep us out of harm's way. Airing November 6, 2013 at 9 pm on PBS Aired November 6, 2013 on PBS

Program Description
Transcript
Making Stuff Safer
PBS Airdate: November 6, 2013
DAVID POGUE: Civilization is built on the human drive to invent. We take the raw stuff of our planet, the materials that give names to the ages—stone, bronze, iron and more—and craft them into new forms, expanding our horizons, exploring hidden worlds and engineering life-changing technologies, always pushing the limits to be colder, faster, safer, wilder. And now a new era is upon us. I'mâ¦
Fire! Fire!
â¦and I'm on a quest to live on the edge and tame our dangerous world. But it's not easy! Think of what Mother Nature throws at us. Is there a way to meet her threats with new technologiesâ¦
Wow, in about two seconds that thing was out!
â¦including buildings that can stand up to a shakedown?
JOHN VAN DE LINDT (Colorado State University): Wow!
DAVID POGUE: And what about threats that seem totally unnatural?
This is uranium?
Can we design defenses to thwart the bad guysâ¦
MICHAEL SOSSONG (Decision Sciences International Corporation): There's something high density in there.
DAVID POGUE: â¦and protect innocent lives?
So this plant can sniff bombs now?
Can engineers protect us from ourselves, with cars that can read the road?
It's not slowing down very much at the curve!
COACH: (Keep your head up! Keep your head up!)
DAVID POGUE: Can we make better gear for our kids?
EVAN BREEDLOVE (Purdue University): That allowed us to see how hard the hits are.
TOM TALAVAGE (Purdue University): We're here to make the game safer.
DAVID POGUE: We'll never live in a risk-free world. And who'd want to?
Are you saying that human trials are beginning right now?
PAULA HAMMOND (Massachusetts Institute of Technology): Here we go!
DAVID POGUE: But we might be able to breathe a bit easierâ¦
Whoa, that was fast!
â¦by Making Stuff Safer.
In Northern California, Stanford University professor Chris Gerdes is hard at work in his favorite lab—the race track—trying to make cars safer.
I hear you are messing around with automotive safety issues.
J. CHRISTIAN GERDES (Stanford University): Trying some new ideas.
DAVID POGUE: Sorry, I couldn't hear you; a racing car just went by.
It looks like he's enlisted the help of an unflappable driver, who never flinches in the face of danger. He's really pushing that car to its limits! I'm dying to meet this intrepid maniac, who seems willing to do anything in the name of science.
Hey, that was reallyâ¦hey, come on! There is no driver! What have you done? What kind of satanic ritual is this?
CHRIS GERDES: This is Shelley, our self-driving Audi TTS.
DAVID POGUE: Self-driving racing car? That's right. There's no one at the wheel! Shelley drives herself. And even though she's got a lead foot and a sweet tooth for squealing tires, her whole purpose in life, or in silicon, anyway, is to keep human drivers alive. It may sound crazy, but Chris designed this robotic speed demon in order to make our cars safer. After all, we take a calculated risk every time we get behind the wheel. Look at all the terrible drivers out there.
So is there an alternative? What if our cars could drive themselves? That brings us back to Shelley. Turns out, she's not just a piece of amazing engineering. She actually helps Chris study how to push a car to the very limits of physical performance without losing control.
CHRIS GERDES: One of the things that's inspired us is to look at how the very best drivers control the car. So we've looked at racecar drivers who are able to get every bit of the friction between the tire and the road.
DAVID POGUE: Many accidents occur when tires lose their grip, when the forces needed to keep the car on the road overwhelm the force of friction. That can put the car into a spin or make it impossible to steer.
Racecar drivers are masters of the road. They push their cars to the limit, at the very edge of control.
CHRIS GERDES: In racing, you get to the limits of the car's capabilities, because you're trying to be fast. In ordinary driving, you may get to the limits of the car's capabilities simply because you're on an icy road. The exact same physics apply, in these two cases.
DAVID POGUE: So Chris wondered, what if he could transfer the reflexes of a racecar driver into the operating system of a regular car? That would be like giving the ordinary driver a computer co-pilot to take over whenever the car begins to lose its grip. But how would he do that?
His first step was to wire high-performance cars with sensors and have racecar drivers speed around the track, as fast as possible, right up to the point of skidding out of control. The sensors recorded how the pros steer, accelerate and brake.
Then Chris translated that driving prowess into software for Shelley's on-board computer. Her two G.P.S. antennas, accelerometers, gyroscopes and other sensors feed her computer real-time data about position and speed. The computer then sends commands to the steering system, throttle and brakes.
Okay, seeing is believing. Unfortunately, Chris seems to think riding is believing, and he wants me to put my life in the hands of Shelley's computer.
CHRIS GERDES: We're off.
DAVID POGUE: Just like a big expensive toy.
Okay so you'reâ¦
CHRIS GERDES: I'm not doing anything right now.
DAVID POGUE: This is not slowing down very much at the curve!
CHRIS GERDES: There's no need to.
DAVID POGUE: It just drove off the road, dude!
CHRIS GERDES: If you don't go up on the rumble strip then you're slow. Any racecar driver will tell you that.
DAVID POGUE: What a smart little car.
Shelley is constantly adjusting her position on the track. Her adjustments just aren't very smooth! But I guess smooth doesn't matter if your only priority is staying on the road. Fortunately, Chris can take over at any time.
But understanding how the best drivers drive is only part of the equation. Chris needs to know more about how ordinary, accident-prone drivers perform, in order to figure out how a computer might help them out. So he and his team have developed another car.
CHRIS GERDES: Welcome to X1. This is an entirely student-built research vehicle that we're using to develop some of the algorithms for Shelley.
DAVID POGUE: It's cool. It's kind of like a dune buggy effect.
Researchers can test all kinds of driving conditions with the X1, by controlling each wheel, individually, to simulate a change in friction between the tire and the road.
CHRIS GERDES: The rear wheels actually steer out, causing the car to go into a spin, and we watch the reaction of the driver to correct that.
DAVID POGUE: All right, so you're going to take it for a drive?
CHRIS GERDES: No, David, we we'd really like to get your data. So we'd like to wire you up and get you to drive the car.
DAVID POGUE: Oh, so the test subject is me? Shocker!
LENE HARBOTT (Stanford University): I'm going to put some E.E.G. electrodes on your scalp. We can monitor your brain activity, as you drive X1 around the skid pad.
DAVID POGUE: I don't know, I think my brain activity might blow out your equipment.
These electrodes won't reveal what I'm thinking, but they'll record patterns in my brain activity, an indication of how hard my brain is working.
I'll be back.
And now I meet my co-pilot.
HOLLY RUSSELL: (Stanford University): I'll be messing with you a little bit. I designed the experiment that we're going to be doing. And while you're driving, I'll be collecting your data, here, on the computer. Are we ready?
DAVID POGUE: Whenever she wants, Holly can take control of any of the four wheels. Any moment now, she'll make the X1 behave as though we've hit a patch of ice.
The squealing is okay?
HOLLY RUSSELL: Yeah, you might want toâ¦oooh. Okay, there you go.
CHRIS GERDES: Not bad.
LENE HARBOTT: This is where Holly triggered the rear wheels to go through a very sharp angle change, to make you feel like you're starting to spin out.
DAVID POGUE: There's an abrupt change in my brainwaves, suggesting I've become super-focused on controlling the car; so focused that if this were a real life situation, I might not notice something as obvious as an oncoming bus.
This data could lead to cars that know just when to step in to bail human drivers out of hairy situations. Which reminds me, I have one final question about self-driving Shelley: how does she stack up against a real pro?
Let's find out. David Vodden has 50 years of experience and knows this track inside and out. But to set a high benchmark, I'm going to take the first lap.
DAVID VODDEN (Thunderhill Raceway Park): Good exit. Good line.
DAVID POGUE: Come on, baby, come on. Give me some horses!
CHRIS GERDES: Two minutes, fifty-one seconds. You've set the mark for this race, and we'll see how the others do.
DAVID POGUE: Woohoo! Two-fifty-one! I'd like to see a piece of software beat that!
The time to beat is two fifty-one, old man.
DAVID VODDEN: Old man?
DAVID POGUE: A professional driver relies on experience, intuition and the feel of the road. He's always thinking ahead.
CHRIS GERDES: This is the warm-up lap.
DAVID POGUE: This is his warm-up lap?
He pushes the car to its limits and smoothly reins it back in.
CHRIS GERDES: Two-nineteen-point-three.
DAVID POGUE: Two-nineteen?
CHRIS GERDES: He's a little bit faster—by more than thirty seconds.
DAVID POGUE: 'Cause you're using a phone instead of a real clock, that's why!
Now Shelley's handlers prep her for the big moment.
PAUL: Start the model.
JOE: Model started.
PAUL: You are now able to exit the vehicle.
DAVID POGUE: Unmanned vehicle on the track!
Shelley makes 200 calculations a second to determine her correct speed and brake-point, based on the available friction.
Robotic, ghost-driven vehicle!
When our cars are this smart, we'll be a lot safer!
So who won, man or machine?
CHRIS GERDES: In third place, with a time of two-fifty-oneâ¦
DAVID POGUE: In second place, with a time of two minutes, twenty-one seconds, Shelley; and today's winner, with a time of two minutes and nineteen seconds: David Vodden.
Today, man beat machine by just two seconds. But Chris is confident that Shelley will beat even the best of us someday soon and make the cars we drive much safer. That is, if we manage to hang onto our spot behind the wheel at all.
It may seem like the stuff of a science fiction movie—just tell your car where you want to go and let it do the driving—but that day may not be far off. Google already has a fleet of self-driving cars that have logged several hundred-thousand miles on surface streets and freeways. They're already officially street-legal in California, Nevada and Florida. Someday, robotic cars may virtually eliminate traffic accidents. But for now, driving is an unavoidable risk that most of us accept, even though some 35,000 people a year will die on the road in the United States.
Flying is much safer. Too bad we have to put up with what happens before takeoff. The frequent flier in me wants to know. Isn't there a better way to combat the risk of terrorism that's quicker and less intrusive?
Now, the Department of Defense is funding a promising and unusual new explosives detection system. It's the brainchild of professor June Medford, at Colorado State University.
JUNE MEDFORD: (Colorado State University): They actually don't need wires, they don't need batteries, and they don't need electricity. And we'll take you down to the deep part of the basement to see them.
DAVID POGUE: This must be some serious ultra-top-secret tech!
JUNE MEDFORD: So, right, ready? Here they are.
DAVID POGUE: What? Behind the salad bar?
JUNE MEDFORD: No. These are our bomb detectors, the plants.
DAVID POGUE: Come on! It's a salad green!
JUNE MEDFORD: It's a plant.
DAVID POGUE: It can't move, it can't talk, it can't touch anything, or, smell anything.
JUNE MEDFORD: But it's got to breathe, just like you and I.
DAVID POGUE: And like us, plants can sense all sorts of things in their environment: light and moisture, temperature and touch, and chemicals, like nitrogen or phosphorous.
JUNE MEDFORD: They have ways to sense and respond to their environment continuously, 365 days a year, 24 hours a day.
DAVID POGUE: Yeah, but they're slow.
JUNE MEDFORD: They're not slow. Take, for instance, the Venus flytrap. It's fast enough that it can catch a fly.
DAVID POGUE: Snap! Whoa. Okay. That was fast.
June's plants can't catch flies, but they can detect traces of chemical explosives through microscopic receptors on the surface of their leaves, stems and roots.
If I could get in there with a microscope, what would these receptors look like? Are they little Velcro or hands?
JUNE MEDFORD: They're little proteins. We actually have a model of what one would look like, like, right here.
DAVID POGUE: Receptors are proteins that are shaped to bind, like a lock and key, to specific chemicals found in nature. But amazingly, some receptors on June's plants can latch on to manmade compounds.
Okay, I'm floating through the air. This is a particle of�
JUNE MEDFORD: Of bomb stuff.
DAVID POGUE: And it's wafting through the air?
JUNE MEDFORD: Wafting through the air. And you can't hide, and it comes in and binds to this.
DAVID POGUE: You've engineered this protein, this receptor, on this plant, to look for something new, a bomb material or explosive, that nature didn't, didn't breed?
JUNE MEDFORD: Absolutely.
DAVID POGUE: Are you genetically modifying that plant?
JUNE MEDFORD: Yes we are.
DAVID POGUE: June works with a group at the University of Washington that custom-designs new proteins. They tailor-made a plant receptor that binds to compounds in explosives.
Then they came up with the sequence of D.N.A., the gene that tells the plants to grow this receptor. And how does June get that D.N.A. into her plants? It's as easy as taking a bath.
JUNE MEDFORD: If you wanted to put a new gene in a plant, all you have to do is take your plants, turn them upside down in the solution, and give them a gentle swirl.
DAVID POGUE: What?
JUNE MEDFORD: Maybe some music.
DAVID POGUE: Come on, that's like a B-movie version of genetic modification. What about the, injector, syringe and the microscope andâ¦
JUNE MEDFORD: It's this.
DAVID POGUE: This solution contains bacteria that naturally infect plants. June inserted the new gene into the bacteria. Now, she leaves it up to them to do the dirty work of transferring the gene into the plant's D.N.A.
That can't work.
JUNE MEDFORD: Yes, it can. Here.
DAVID POGUE: That's like a kindergartener's science project.
JUNE MEDFORD: Here, you do it, David.
DAVID POGUE: Just by swishing it around upside down?
JUNE MEDFORD: Indeed, indeed. Think about plant sex. Slow, slow. There you go.
DAVID POGUE: Alright, I've done it. So this plant can sniff bombs now?
JUNE MEDFORD: The seed from that plant can sniff bombs.
DAVID POGUE: Oh, the seed. So this, this was the plant sex, and the offspringâ¦
JUNE MEDFORD: The offspring will sniff bombs.
DAVID POGUE: â¦will have the superpower.
The swirled plants eventually flower and produce seeds that June grows in Petri dishes. These tiny plants are bomb detectors, but how, exactly, can they tell us there's a bomb nearby? It's not like they can wag their tails and bark.
When molecules of explosives bind to the engineered receptors on the surface of the plant they trigger a powerful response.
And, what exactly, happened?
JUNE MEDFORD: Well, they detected a substance. It activated a histidine kinase signal transduction pathway that sent a high energy phosphate bond from a histidine to aspartate, leading to a conformational change in the protein, activating our degreening circuit, leading to loss of chlorophyll.
DAVID POGUE: What does that mean?
JUNE MEDFORD: The plants turned white.
DAVID POGUE: For now, it takes 48 hours for the plants' leaves to lose all their chlorophyll, the pigment that makes them green. June tracks the progress with time-lapse cameras. But she's working on reducing that time to just seconds. Fast as a Venus flytrap.
So, someday, this could be the front yard at the airport.
JUNE MEDFORD: That's right. This is the entrance to the airport. This is the entrance to the football stadium.
DAVID POGUE: Or a shopping center, theater.
JUNE MEDFORD: Theater. The entrances to any area, including the National Mall, in Washington, D.C.
DAVID POGUE: The only problem is, what happens, if somebody just happens to have planted white flowers? Wouldn't everyone be like, "Aaah! Bombs!"?
JUNE MEDFORD: The leaves are still green, David.
DAVID POGUE: Oh, it's the leaves that change color.
June has harnessed the incredible machinery of nature to create bomb detectors powered by water and sunshine. And now there's another new technology that may protect us against even scarier weapons like a dirty bomb, or an actual nuke.
Every year, about 16-million cargo containers arrive at American ports. The worry is that any one of them might contain dangerous radioactive contraband. Customs officials can scan only a small percentage of them. Like giant Geiger counters, radiation portal monitors can detect the gamma rays emitted by uranium, plutonium and other nuclear materials, but not all our ports are equipped with this gear. And besides, those detectors can easily be fooled. X-rays can spot nuclear materials, but they can be harmful to living things, like port officials.
What if there was a harmless way to see inside every container? It would be like having superpowers.
At the southern California offices of Decision Sciences, Michael Sossong is working on just such a system. But before he shows me how it works he's going to demonstrate the shortcomings of standard radiation detectors.
He has me wield a Geiger counter.
MIKE SOSSONG: The nuclear materials that we're looking for are not the only radioactive things out there. There's a lot of other things that are putting off radiation that we use in our daily lives.
DAVID POGUE: Really? Like?
MIKE SOSSONG: Like bananas.
DAVID POGUE: Bananas?
Mike tells me that bananas actually contain potassium 40—a radioactive isotope—but way too little to hurt us. Still, containers of bananas have set off alarms.
So the, the port inspectors, they'd be looking with their monitors and they'd be like, "Whoa, there's something radioactive in there;" freak out everybody. And then they're like, "Oh, it's just bananas."
MIKE SOSSONG: Right.
DAVID POGUE: Water softener salt contains even more of that potassium, and some ceramics get their bright colors from radioactive glazes.
MIKE SOSSONG: Yes, and that's a, a serious problem with the detectors that we have out there now, is that they're getting hundreds of false positives, at a busy port, a day.
DAVID POGUE: It can take hours to hand-inspect shipments to confirm a false positive, and that's time port officials could use to detect real threats. But there's an even more chilling problem.
MIKE SOSSONG: We actually call this the, the "cube of terror," the type of arrangement that a terrorist might use, trying to smuggle these materials.
DAVID POGUE: It's just three layers of lead inside a steel-lined milk crate. And this is a cube of uranium. Don't worry. This is depleted uranium, not the kind used in bombs. But it's still radioactive enough that I need to wear gloves to handle it.
Oh, man, it's very heavy!
Nuclear materials are dense. This cube weighs nearly 50 pounds.
I'm all set.
MIKE SOSSONG: All right.
DAVID POGUE: Behold: one cube of terror and no radiation to give it away.
MIKE SOSSONG: You could now drive this past any passive radiation detector, and they wouldn't detect it.
DAVID POGUE: Frank! It's just milk! Don't worry about it!
But now there may be a way to peer into the cube. It's a technology based on something from outer space: cosmic rays. When these high-energy particles hit Earth's atmosphere, they create subatomic particles called muons. Traveling at nearly the speed of light, muons rain down on our planet all the time and zip through just about anything in their way, including you, me and shipping containers.
This contraption may look like nothing more than a huge metal frame, but it can detect those muons.
You know it looks like it's nothing but a bunch of aluminum pipes.
STANTON SLOAN (Decision Sciences International Corporation): You're right, it's just aluminum tubes. There's nothing, you know, very exotic in terms of the physical machine. The, there's a lot of software, a lot of complexity in the software.
DAVID POGUE: More than 4,000 aluminum tubes make up this lower detector, and 4,000 more form the upper detector. Each tube has an electrically charged wire running through it. When a muon passes through the tube, the wire sends a signal to a bank of computers. The upper detector records the path of each muon as it enters the scanner. As the muon penetrates the container and its contents, these solid objects change its trajectory a bit—the denser the material, the greater the change. When the muon hits the lower detector, the system records how much the muon's path has changed and calculates the density of every object in the container. But can it look through lead and spot a hidden block of uranium?
This I've got to see.
MIKE SOSSONG: Okay, see that box of office paper over there? Pull that out and make a nice little spot for it.
DAVID POGUE: Michael helps me hide my evil milk crate in a shipment of innocent goods.
So here's where the magic happens. As thousands of muons stream through the detector, the computer builds up an image of the container and its contents.
MIKE SOSSONG: You can already see. There's the pallets; you can see the corner posts of the container. We get a full 3D picture of what's in the container, and that's critical, because it, it helps to address this issue of hiding things behind other, more dense objects.
DAVID POGUE: Materials used in nuclear weapons are among the densest on Earth.
Within seconds the uranium shows up.
MIKE SOSSONG: This isn't just a load of paper and kitty litter. There's something high density in there. And you can actually see the nice red arrow.
DAVID POGUE: That's amazing. So, even though I put that uranium in three half-inch-thick layers of lead and inside the steel of a container, like Superman, with X-ray vision, you are seeing that block.
MIKE SOSSONG: It's actually better than X-ray vision; it's muon-vision.
DAVID POGUE: For a next step, a full-scale scanner is being tested at Freeport, in the Bahamas. If it pays off, this technology may help protect our ports against nuclear threats.
But even the best border security won't protect us from what Mother Nature routinely throws our way! Of all our planet's upheavals, earthquakes are among the most terrifying. We can't predict them, and we sure can't stop them. But, increasingly, engineers are learning how to protect against their awesome power.
That's good news, because one of my favorite cities is also one of our most vulnerable: San Francisco. I'd always thought these skyscrapers would be the hardest hit in a big quake, but, in fact, the taller the building, the more flexible it is. Skyscrapers can sway without falling over.
What we need to worry about are thousands of buildings that went up before the 1970s. They're called "soft story" buildings, because their ground floor is an open space, usually a garage, with large doorways.
In an earthquake, that structurally soft story can't support the motion of the upper floors and it collapses.
In 1989, a magnitude 6.9 earthquake damaged hundreds of these structures in the Bay Area, and left thousands homeless. Today, throughout California's seismic zones, some 2-, to 300,000 people still live in these death traps. They're sitting ducks. The risk is so high that cities across the state are writing laws requiring that these structures be retrofitted. But how can engineers figure out exactly what will work?
Well, for starters, a five-university team built a replica of a soft story building from the 1920s. But they couldn't just sit around and wait for the earth to move. They staged their own earthquake. Then they followed that with a devastating aftershock that brought this building to its knees.
But we're getting ahead of ourselves, because before they flattened it, engineers spent a month testing ways to keep the structure standing.
It's built on the largest outdoor shake table in the world, at the University of California, San Diego. Powered by massive hydraulic pistons, this 172-ton brute can simulate the most devastating earthquakes ever recorded. It can also make a mean milkshake.
Seriously, for team leader John van de Lindt this is the project of a lifetime.
JOHN VAN DE LINDT: This is the first time a building this size, that represents a true soft-story building, has been tested.
DAVID POGUE: His team will test four engineering solutions that performed well in computer models.
JOHN VAN DE LINDT: We're looking at design and retrofit methods to try and make these structures safer for the many, many families around the United States that live in them.
DAVID POGUE: The first retrofit involves panels of a new building material called "cross laminated timber," or C.L.T. C.L.T. is made of lumber, stacked at right angles and glued together, which makes for panels that are very strong and stiff.
Two sets of panels are placed on the garage floor and anchored to the ceiling. During an earthquake, they should rock in place and help absorb energy from the motion of the upper stories. But the C.L.T. panels aren't bearing the burden alone. They're attached to steel rods that run straight down into the foundation.
JOHN VAN DE LINDT: The steel rods, they have to transfer forces from the cross-laminated timber, while it rocks, and this will dissipate the energy from the earthquake.
DAVID POGUE: Before the test, they fully wire the place: 30 cameras and 300 sensors to measure the movement and behavior of each part of the building.
Now it's time to rock and roll.
DAN RADULESCU: Loma Prieta, in 10, 9â¦
DAVID POGUE: This will be a simulation of the 1989 earthquake that took 63 lives and destroyed countless structures. But with its retrofit in place, this building's ready to rumble.
DAN RADULESCU: Three, two, one, running.
DAVID POGUE: Can just a few wooden panels really prevent collapse? The original earthquake lasted about 15 seconds. That may not sound like a long time, but if you happen to be inside a building that's about to collapse, it must feel like an eternity.
Cameras reveal the C.L.T. panels rocking in place. At last the test is over. They head inside and look for damage.
It's clear that the panels saved the day, but the rocking motion put so much stress on the steel rods that they actually stretched.
JOHN VAN DE LINDT: All in all, this test was absolutely perfect, I'd say phenomenal. If this wasn't here, the building would have collapsed.
DAVID POGUE: The researchers document every crack and break. Some repairs would be needed, but the building would still be livable.
C.L.T. panels are the simplest of the retrofits they'll test. Steel frames strengthened the openings on the ground floor and performed even better than the C.L.T. panels, allowing minimal damage to the building. When engineers added plywood reinforcement on the upper floors, the steel frames kept the structure virtually intact.
Same goes for the fourth retrofit: high-tech dampers that acted like shock absorbers on the bottom floor and kept the building standing.
All in all, four for four.
JOHN VAN DE LINDT: We tested four retrofits to this point. They've done exactly what we wanted them to. Sometimes even performed a little bit better than expected. All these retrofits are a viable option.
DAVID POGUE: That's great, but what would be the point of building a four-story structure on a shake table and then letting it stand?
So now, with all the retrofitting removed, there's one last test to run. Engineers have modeled this one in the computer, but they've never actually seen a soft story building collapse, until today.
JOHN VAN DE LINDT: So the two safe areas areâ¦is back behind the red tape there, and then you can walk around the building, and you'll see red tape over here.
DAVID POGUE: For earthquake engineers, this is an epic event.
They start out with a few gentle shakes, then they gun it, hitting it with equivalent of a 6.7-magnitude shake. They watch as the second floor juts out over the garage level by nearly a foot and a half. The engineers are shocked to see that it's still upright.
JOHN VAN DE LINDT: Wow, so 17 percent drift and it's standing. I guess that answers the question on how much drift these can take.
DAVID POGUE: What will it take to bring this baby down? They hit it once again with a large aftershock.
DAN RADULESCU: One hundred percent M.C.E. level, in 3, 2, 1, running.
JOHN VAN DE LINDT: Wow. Unreal. Wow. I don't think I've seen something like that.
DAVID POGUE: The building they worked so hard to protect is just a pile of lumber, beyond repair.
JOHN VAN DE LINDT: I do have a soft spot in my heart for the building. But I know what we've done will provide engineering and science what they need to make these types of dangerous buildings much safer for the families that live in them.
DAVID POGUE: Let's hope Californians apply those retrofitting techniques before the "Big One" hits.
Luckily, most of us don't live in earthquake country, but none of our homes are beyond the reach of a far more common threat: fire.
Over the past century, death rates from fires have dropped 90 percent in the United States, mostly thanks to smoke detectors, fireproof materials and fire codes. Still, nearly 3,000 people die in residential fires each year. And now the risk of wild fires seems to be on the rise, most likely due to higher temperatures and droughts, brought on by climate change.
We have foams and chemical retardants to knock down flames and slow the spread of fire, but many of these products are toxic to plants and animals. Short of installing sprinkler systems throughout the wilderness, can't we find a better way to combat fires?
I've come to the headquarters of EarthClean, in Minnesota to find out.
Rumor on the street is you've come up with something better than water for putting out a fire.
SCOTT BOCKLUND (TetraKO/EarthClean Corporation): This is going to put the fire out faster than anything you've seen before.
DAVID POGUE: Vanilla pudding?
This goop is called TetraKO, and it's different from plain old water in an important way.
SCOTT BOCKLUND: The unique thing about it is it actually adheres to where it's sprayed. You can spray it on a ceiling, you can spray it on a wall, and it would actually stick and stay there.
DAVID POGUE: So the, the firefighter, if it were water, would have to stand there hosing, hosing, hosing, but now can just spray the goop, and then move on to the next wall?
SCOTT BOCKLUND: Exactly.
DAVID POGUE: But won't this gel just melt away in a fire?
SCOTT BOCKLUND: I'll show you that the stick does actually burn.
DAVID POGUE: Apparently, this stuff can also keep things from going up in flames.
So, what's in there, exactly?
SCOTT BOCKLUND: Well, our main ingredient is cornstarch.
DAVID POGUE: Cornstarch?
SCOTT BOCKLUND: If you'd like to try it, you can.
DAVID POGUE: Wait, what are you doing?
SCOTT BOCKLUND: It's all natural. You can eat it.
DAVID POGUE: So how does cornstarch stop fire?
ERIC MORRISON (TetraKO/EarthClean Corporation): So, you've all made gravy. So here's my cornstarch.
DAVID POGUE: Just add a little water, apply some heat, and that simple white powder becomes white goo.
ERIC MORRISON: This will thicken up, as soon as it gets hot.
DAVID POGUE: Cornstarch consists of long chains of glucose—a form of sugar—tightly packed into tiny granules. When mixed in water and heated, those chains separate. They swell and create a net-like structure that makes the mixture thicker. That's why we use corn starch to thicken gravy, and it's why this stuff sticks to walls in a fire, instead of melting.
So, next time I'm making Thanksgiving dinner, and it bursts into flame, as typically happens with me, what should I grab to throw on it? A bucket of water or the pot of gravy?
ERIC MORRISON: Pot of gravy.
DAVID POGUE: The gel also contains polymers, long molecular chains, dissolved in water, which give it the special properties of a non-Newtonian fluid.
Most fluids are just plain Newtonian. Water is the classic example. Stir it, whisk it, shake it as much as you want and it doesn't change its thickness. Juice, honey, and most oils are also Newtonian fluids. But non-Newtonian fluids change when you apply force to them.
The best-known non-Newtonian fluid is ketchup.
ERIC MORRISON: And ketchup has this property. When it's not being squeezed, it's a solid. But if you shake it up and it starts to move around, it liquefies.
DAVID POGUE: So this is a, a facial cleanser. It's a gel, but it's pretty solid.
ERIC MORRISON: It is a solid.
DAVID POGUE: The bubbles are frozen in space. And yet, through the miracle of non-Newtonian fluids, it turns into a liquid?
ERIC MORRISON: Exactly.
This is also a solid.
DAVID POGUE: And this is hair styling gel.
ERIC MORRISON: And if we pump it, it liquefies.
DAVID POGUE: It turns into a mist.
ERIC MORRISON: Right.
DAVID POGUE: The non-Newtonian firefighting gel also has a split personality.
So the TetraKO is a gel, when it's in the fire truck, and then you're spraying it, it comes out of the nozzle, instantly turns into a liquid, so it can spray. Then it lands on the wall and then what?
ERIC MORRISON: Turns back into a solid.
DAVID POGUE: Oh, my gosh!
But fire on a little paint stick is one thing. Let's see how the corn starch does at putting out a real fire, like my little fake apartment here.
The Fire Department of White Bear Lake, Minnesota helps us stage a test.
The gel hits the burning surfaces and sticks, smothering the flames. The cornstarch thickens the gel and releases clouds of steam.
Wow. About two seconds, that thing was out, and I mean completely out.
Local fire departments are using this new firefighting gel in the field. And though it costs more than just spraying water, some think it's worth it.
Chief Zikmund, can I talk to you a sec?
NYLE ZIKMUND (Spring Lake Park-Blaine-Mounds View Fire Department): You bet, glad to.
DAVID POGUE: Have a seat.
NYLE ZIKMUND: Thanks, I'll pass.
DAVID POGUE: So, you've used this stuff in real fires, out in the real world?
NYLE ZIKMUND: Out in our community, we've had it on a half-dozen fires. The crew would hit it from the doorway, and the fire's out, and it stays out. This is some of the best technology I've seen come along in years.
DAVID POGUE: TetraKO is in beta-testing for use in wildfires. It can be sprayed on vegetation and buildings in the path of an approaching fire, up to a day in advance. And it's non-toxic, safe for the environment, wildlife and us.
We've made great strides in protecting ourselves from fires, but what about protecting ourselves from the fires that flare up in our bodies? I'm talking about infectious diseases.
Vaccines are one of our greatest weapons against these deadly enemies. They've saved millions from polio, smallpox, measles, whooping cough and dozens of other illnesses. But delivering vaccines can be a challenge. Many need to be kept cold, and sometimes that's nearly impossible. And most require an injection. Where medical supplies are lacking, needles are sometimes re-used, which can spread disease. Bottom line: millions of people are not getting the vaccinations they need.
But I hear there's a team at M.I.T. working to overcome these obstacles. Scientist Pete DeMuth is developing a vaccine patch smaller than a dime.
So, what does this patch look like? It exists?
PETER DEMUTH (Massachusetts Institute of Technology): Yeah, it exists. You can take a look at a couple right here. And these needles are actually on the order of half a millimeter or less in length.
DAVID POGUE: So that'd be like this?
PETE DEMUTH: Exactly.
DAVID POGUE: And how many are there?
PETE DEMUTH: So, this patch actually has about 100 needles, but patches are made where there can be, even, thousands of needles.
DAVID POGUE: Looks harmless until you see it under the microscope.
PETE DEMUTH: All right, and then we'll bring this into focus.
DAVID POGUE: Whoa! Evil! Looks like a medieval torture device!
The next step is to coat these needles with fine layers, or films, that contain the vaccine. But how do you layer anything on something this small? You've got to know how to work at the nano-scale, down at the molecular level, like Professor Paula Hammond.
PAULA HAMMOND: Well, this is actually where we build the films that contain the vaccine.
DAVID POGUE: Hi, how are you doing?
PAULA HAMMOND: Yes, yes, we love our friend here.
DAVID POGUE: This is it, huh?
PAULA HAMMOND: This is it.
DAVID POGUE: A robotic arm dips the microneedle patch into trays containing the vaccine's components.
You, sort of, co-opted the ancient principle of chocolate fondue.
PAULA HAMMOND: The way that we make the coating, is that we take the components that make up our vaccine; one of the components is negatively charged, and the other component is positively charged.
DAVID POGUE: The microneedle patch is negatively charged. First, it's dipped in a positively-charged component and a microscopic layer forms. Then, it's dipped in a negatively-charged component, and a new layer forms. In this way, dozens of layers can be built up on the microneedles and then embedded in the skin, like a tattoo. Over days or weeks, they dissolve and release the vaccine.
But what are these components?
PAULA HAMMOND: The positively-charged ones are biodegradable polymers, in this case, plastics. And the negatively-charged ones are D.N.A.
DAVID POGUE: Wait, so, there's a polymer, and there's D.N.A., which is genetic material. I thought you said it's a vaccine.
PAULA HAMMOND: Actually, D.N.A., in our case, is the vaccine, which is very unique but also very powerful.
DAVID POGUE: Most vaccines are created from weakened or killed microbes, or from parts of microbes. The immune system mounts an attack, even though there's no real threat. Some immune cells remember the episode. Should they ever encounter the actual microbe, they'll spring into action and destroy the invader.
A D.N.A. vaccine doesn't deliver any bits of microbe. It delivers a stretch of D.N.A. that contains a gene for a bit of microbe, a protein. This gene inserts itself into our D.N.A. Now, our own cells produce proteins that trigger an immune response.
And the D.N.A. requires no refrigeration.
PETE DEMUTH: Okay, David, are you ready?
DAVID POGUE: Dude! In what universe is that a microneedle?
PAULA HAMMOND: We don't have to deal with this old technology anymore.
DAVID POGUE: Oh, that's the before, and this is the after.
PAULA HAMMOND: That was before, yeah.
DAVID POGUE: You guys are just a laugh riot.
PAULA HAMMOND: And I'm just going to apply this to your wrist.
DAVID POGUE: So, this is human trials, starting right now?
PAULA HAMMOND: Well, let's see how it goes.
All right. And we're going to place this right here.
DAVID POGUE: Ow!
It doesn't hurt at all.
PAULA HAMMOND: Just a little pressure.
DAVID POGUE: Like you're holding up a plastic game piece to my skin.
PAULA HAMMOND: It's really nice, because these microneedles are too short to reach the nerve endings in your skin.
DAVID POGUE: And, this particular one, what nasty D.N.A. is it injecting into my body?
PAULA HAMMOND: We didn't put anything on yours. This is just a blank to give you an idea of what the experience will be like.
DAVID POGUE: Wow. I don't see blood; I don't see shredded flesh, just that little ring.
PAULA HAMMOND: That's right, exactly.
DAVID POGUE: So, not only does this eliminate the problem with keeping the vaccine cold all the way from the manufacturer, not only does it solve the problem of needles being reused or misused, but it also doesn't hurt. That, you could argue, is an advantage over huge nasty pieces of metal.
PAULA HAMMOND: It's a huge advantage: no more crying children. You have happy parents, as well.
DAVID POGUE: Although, the one downside, I'd have to say, is that you don't get the little lollipop at the end for your suffering.
PAULA HAMMOND: We would like to give you a little lollipopâ¦
DAVID POGUE: Aren't you sweet?
PAULA HAMMOND: â¦for being such a great sport.
DAVID POGUE: It's a polymer!
This radical new vaccine system is still in the testing phase. If it lives up to its promise, it could eventually save millions of lives around the world.
In our pursuit of safety, we've pushed the limits of science and technology and come up with amazing innovations, and yet, we keep on doing dangerous things, sometimes just for the thrill of it. Hey, I've even pulled a few crazy stunts in the name of science! But sometimes, we're just not aware of the real risk. Take football: it's more than a sport. It's a part of American culture and a passion for millions of fans. Part of its appeal is that it's a serious contact sport.
But over the past decade, there's been a spate of stories about veteran football players succumbing to depression and dementia. And several top players have committed suicide. Autopsies of their brains revealed that an abnormal protein had destroyed cells in areas that regulate impulse control, judgment and memory. The condition appeared to be caused by a history of multiple concussions.
A concussion occurs when a violent blow to the head or sudden acceleration, like whiplash, forcefully jars or shakes the brain. Symptoms can include an inability to think clearly, blurry vision and dizziness. But scientists still don't understand exactly what happens to the brain in a concussion.
Four years ago, neuroimaging specialist Tom Talavage and his colleagues at the Purdue Neurotrauma Group began tracking football players at local high schools, because professional players are not the only ones whose brains take a beating.
BRANDON GAWLIK: I had two concussions: one in eighth grade and one in sophomore year, so, last year.
VINCE MIRELEZ: I just felt really dizzy. I felt sick to my stomach.
ALEX JONES: But I didn't think anything of it, and I went back in and played.
DAVID POGUE: The research team wired players' helmets.
EVAN BREEDLOVE: This is our sensor package, and it fits in between the padding and this space here.
DAVID POGUE: These accelerometers record the intensity and location of each hit, as well as the motion of the player's head. A wireless connection sends information to the researchers in real time.
EVAN BREEDLOVE: This may be a hit of the forehead against the helmet.
DAVID POGUE: Later, the data is translated into a plot.
EVAN BREEDLOVE: You can imagine unzipping the helmet in the back and pulling it open and flattening it out. Blue dots represent small hits. Yellow and red dots represent big hits.
DAVID POGUE: Before the season began, the researchers compiled a baseline cognitive score for each player, measuring things like attention span, reaction time and problem solving. And a functional M.R.I. scan measured brain activity, while the player performed another set of tests. The plan was to run the same battery of tests each time a player sustained a concussion.
COACH: Are you dizzy? Sick to your stomach?
DAVID POGUE: After two weeks of practice and two weeks of games, the players had sustained plenty of hits, but no one suffered a concussion.
COACH: Everything looks good.
DAVID POGUE: So the researchers retested several players anyway, expecting little to no change from their baselines. The results stunned them.
TOM TALAVAGE: This was a player who was never diagnosed with any sort of concussion. There's no reason that an athletic trainer or a coach or any other player would have ever recommended that he be examined.
DAVID POGUE: Yet his M.R.I. showed his brain working much harder on the same tests he'd aced four weeks earlier. He also showed cognitive deficits, especially in his visual memory. No concussion, but he had sustained several hundred hits to the head.
EVAN BREEDLOVE: This looks like a player who's getting their head down, charging into the line, and takes a hit every single play.
DAVID POGUE: Those hits produced the kind of impairment the research team had assumed would only be caused by concussions. And this was just one player of several with the same impairment.
Twelve weeks after the season ended, the players were tested again. Their scores returned to normal, but the surprising, earlier results forced the scientists to rethink assumptions about head injuries.
TOM TALAVAGE: What we're only starting, now, to understand, is that the players who are getting hit a lot are actually ending up just as, if not more impaired as the players who get concussions.
DAVID POGUE: Some 3,000,000 kids, under the age of 18, play football in the United States. Could repeated blows to the head, even ones that don't qualify as concussions, affect their academic performance? And just how great is the risk of permanent damage for these kids and kids playing other contact sports?
TOM TALAVAGE: These are young kids, their brains are still developing. If we are causing some low level of damage, the body is going to be spending time repairing those injuries. So, as these kids are playing more and more football, are we going to find that their brains are becoming less and less normal?
DAVID POGUE: Tom and his colleagues hope their research will answer that question. In the meantime what can we do to make young athletes safer? If we can't get them to give up football for basket-weaving, can we at least build a better helmet?
Traditionally, football helmets have been designed to prevent skull fractures, and they do that remarkably well. But how can we protect the brain inside the skull during head-on collisions and other blows?
ERIC NAUMAN (Purdue University): Every hit, whether it's from the side or the front or the top, is also going to cause the head to rotate, either back and forth, or side to side, or even in this twisting motion, or some combination of those.
DAVID POGUE: These rotational forces can be just as devastating as direct hits. So, Eric Nauman is developing a next-generation helmet, based on a double shell design. Separated by a layer of foam, the two shells move independently. In an impact, the outer shell should absorb some of the rotational force before it reaches the brain. It will take years of testing to reveal whether this new helmet can actually protect young brains during routine play.
The message about head injuries and brain damage is also reaching the playing field.
COACH: Head up! Head up! Head up!
DAVID POGUE: Some coaches have begun to emphasize proper tackling techniques, where players lead with the shoulders and get their heads out of the way.
TOM TALAVAGE: We certainly see that players who take fewer hits to the head or fewer large hits to the head tend to show fewer changes in their neurocognitive testing, in their neuroimaging. With better monitoring, better equipment and better technique, we're here to make the game safer.
DAVID POGUE: When it comes to safety, most of us think of children first and foremost. They're our future; who could blame us for trying to protect them from every peril? If we could, we'd probably dress them in bubble wrap and childproof the entire world.
But I wonder: is there such a thing as too safe? Don't we all need to test our physical limits? Isn't that how we gain the confidence we need to explore, to invent and create the innovations that improve our world? Nothing ventured, nothing gained!
Maybe the key to living well is finding the sweet spot between an acceptable level of risk and making stuff safer!
Broadcast Credits
Jill Shinefield
Joseph Kleinman
Minna Kane
Olga Arana
Peter Bonilla
Dustin Eddo
Alexandra Gallo
Chris Kellett
Ken King
Drew Levinson
Dean Miller
Patrick Schaefer
John Toffolo
Warren Wolfe
Brian Funck
Kevin Purcell
Cindy Love
Corbis
Department of Defense/US Customs
Framepool
Getty
Oddball Film + Video
T3Media
The WPA Film Library
UWTV
VA Boston/ Boston University
Robert Beckley
Defense Threat Reduction Agency
Department of Homeland Security Science and Technology Directorate
Kevin Tod Haug
Institute for Protein Design, University of Washington
Ioana Patringenaru
Dan Radulescu
Jim Schenke
Smith Memorial Playground & Playhouse
John Verrico
Tony DeRose
Dale Dougherty
Alex King
Tim McGee
Amy Moll
Mike Petrich
Ainissa Ramirez
Thea Sahr
Eric Siegel
Stephen Streiffer
Desney Tan
Jessika Trancik
Thomas Tretter
Phil Weilerstein
John Luker
Musikvergnuegen, Inc.
Rob Morsberger
Eddie Ward
Janice Flood
A NOVA Production by Gemini Productions LLC for WGBH/Boston
© 2013 WGBH Educational Foundation
All rights reserved
This program was produced by WGBH, which is solely responsible for its content.
Image:
- Image credit: (David Pogue)
- Courtesy David Pogue
Participants
- Scott Bocklund
- TetraKO/EarthClean Corp.
- Evan Breedlove
- Purdue University
- Pete DeMuth
- Chris Gerdes
- Stanford University
- J. Christian Gerdes
- Stanford University
- Paula Hammond
- Lene Harbott
- Stanford University
- June Medford
- Colorado State University
- Eric Nauman
- Purdue University
- Holly Russell
- Stanford University
- Stanton Sloane
- Decision Sciences
- Michael Sossong
- Decision Sciences
- Tom Talavage
- John Van De Lindt
- Colorado State University
- David Vodden
- Thunderhill Raceway
- Nyle Zikmund
- SBM Fire Department
Preview | 00:30
Full Program
Notice: Undefined index: video_length in /home/nova/zend/application/templates/resources/multi-ep-pro.phtml on line 500
Full program available for streaming through ...
Watch Online
Full program available
Soon