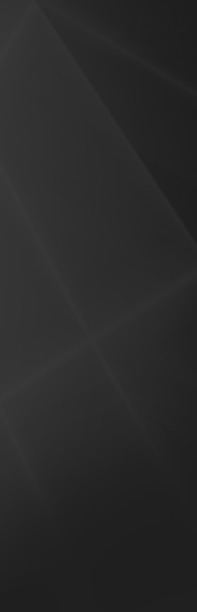
Deadliest Earthquakes
Big quakes are inevitable, but can we lessen their devastation? Airing June 25, 2014 at 9 pm on PBS Aired June 25, 2014 on PBS
- Originally aired 01.04.12
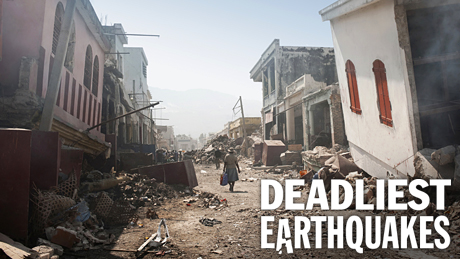
Program Description
Transcript
Deadliest Earthquakes
PBS Airdate: January 11, 2011
NARRATOR: In 2010, massive earthquakes rattle the globe. In Haiti, over 200,000 people are killed. Weeks later, cities half a world away, in Chile, are leveled, half a million buildings damaged.
Around the globe, millions live above active earthquake zones. What causes these earthquakes? And why are the subterranean tremors so hard to predict?
BILL MCGUIRE (University College London): We can't see earthquakes coming. That's the problem. One minute, they're not there, the next minute: wham!
NARRATOR: Now, the race is on to find ways to warn us of impending disaster. And science is revealing new insights into what is actually going on beneath the surface.
KEN CREAGER (University of Washington): It tells us something about the way plates slide past each other that we had no clue about before.
NARRATOR: But the clock is ticking. And the next one could be in our own backyard.
TOM JORDAN (Southern California Earthquake Center): This is earthquake country. We don't know when that earthquake or sequence of earthquakes will occur, but they're inevitable.
NARRATOR: The earth-shattering truth behind The Deadliest Earthquakes, next on NOVA.
Port-au-Prince, Haiti; January 12, 2010; 4:53 p.m.: The city is hit by the western hemisphere's deadliest earthquake in a century. This busy metropolis is reduced to rubble in less than a minute. Two hundred thirty three thousand people lie dead.
Millions more are injured or orphaned.
As 2010 unfolds, more earthquakes strike around the world. Each time, there is no warning and often massive loss of life.
Scientists are working around the clock to unlock the mysteries of earthquake prediction. Within days of the Haiti catastrophe, Professor Eric Calais, a seismologist from Purdue University, arrives on the island.
Calais and his colleagues are at the cutting edge of earthquake research. They could see this disaster coming. They'd known for years that a Haitian earthquake was inevitable. Today, Calais is leading a rapid response mission to find out exactly what happened and to assess the risk of more quakes.
For nearly a decade, he's used G.P.S. markers scattered throughout Haiti to monitor the ground shifting under the island.
ERIC CALAIS (Purdue University): We're headed to a place where we have what we call a benchmark. We have a precise position for that benchmark before the earthquake, and now we're going to measure its position after the earthquake.
NARRATOR: Many markers are placed in well-built police stations. Embedded in solid concrete roofs, they survive the earthquake.
ERIC CALAIS: The benchmark that we're talking about is this. It's not spectacular at all. It's a piece of metal, stainless steel, that we sealed at the top of this building. And there is a little dimple that's about a millimeter wide. And this is the point we've been tracking over time, since 2003, the first time we measured this point.
NARRATOR: Calais' G.P.S. measurements reveal telltale movements of the earth. Over a decade, the satellites tracked the ground south of Port-au-Prince creeping east and the ground north of the city creeping west, at fractions of an inch a year. Then, during the quake, a huge slippage takes place.
ERIC CALAIS: This location, here, moved by about 30 centimeters during the earthquake. So, this is 30 centimeters, more or less like that. So this whole area here has moved 30 centimeters to the east during this earthquake.
NARRATOR: These movements are a clue to what happened underground. The earth ruptured nearly a foot along a hidden fault line. Most earthquakes originate in deep fractures in the crust, miles beneath the surface. These giant fractures crisscross the globe, splitting the planet's 50-mile thick crust into around a dozen huge rocky slabs.
BILL MCGUIRE: The earth is enclosed in a rigid shell, if you like. This shell is made of different plates, rigid rocky plates, which move around at about the same speed as your fingernails grow. There's this, sort of, dance of the plates going on, all the time, around the planet's surface.
NARRATOR: Geologists call this dance, "plate tectonics." The planet's internal heat moves the huge plates. Above the hottest zones, molten rock rises and solidifies, creating new crust. The new crust jostles for space, forcing crust on cooler edges to grind against other plates or push beneath them.
But wherever or however plates collide, they generate earthquakes.
BILL MCGUIRE: Earthquakes happen because these huge chunks of rock that form the earth's crust don't slide against one another nice and smoothly, they stick and they lock. So you're getting this enormous accumulation of strain, and then you're getting it released, in a matter of seconds, during the earthquake.
NARRATOR: The quakes can originate tens of miles down, but the energy they generate creates deadly vibrations on the surface.
BILL MCGUIRE: The amount of energy generated in the biggest earthquakes are comparable to many, many thousands of nuclear bombs going off. There's just a huge amount of energy, absolutely vast.
NARRATOR: Haiti lies directly above a network of massive faults, where the Caribbean plate meets the North American plate. As the plates slowly creep past one another, the rock distorts and stretches like rubber, building up enormous stress.
Since 2003, Calais and his colleagues used ground movement data to calculate stress levels at the plate boundary. Calais realized a deadly quake was inevitable.
ERIC CALAIS: What we saw was a fault being loaded just like a rubber band.
NARRATOR: Simple math allowed him to calculate the precise elastic strain on the fault.
ERIC CALAIS: The last earthquake on that fault occurred about 250 years ago. And it's building up elastic energy at seven millimeters per year. Seven times 250 is about 1.8 meters.
NARRATOR: That's almost six feet of stored strain, equivalent to 100 Hiroshima atom bombs.
Seismologists use a logarithmic scale to measure earthquake strength. Each number indicates a seismic wave 10 times larger than the number below. A two is insignificant but a seven or above can be immensely destructive.
In 2008, Calais announced the Haitian fault could generate an earthquake above magnitude seven. Two years later, his forecast proves accurate.
Calais came closer than anyone to giving an accurate warning of the quake, but like all seismologists, he had no idea exactly when it would happen.
ERIC CALAIS: We cannot predict earthquakes. We had no way of telling whether it was going to be today, tomorrow, in ten years, in a hundred years; so we didn't put a date on it. And it's not that we were afraid to put a date on it, it's that, as a scientist, we can't.
NARRATOR: Today, seismologists can calculate the pressures on the plate boundaries, but they can't predict when the faults will rupture.
BILL MCGUIRE: We can't see earthquakes coming. That's the problem. One minute, they're not there, the next minute: wham!
NARRATOR: Back in the lab, Calais now attempts to find out how much stress remains in the Haiti fault. It's bad news.
ERIC CALAIS: We saw, on January 12, that only a small portion of the fault actually ruptured. The fault itself, from the Dominican Republic all the way to the western end of the southern peninsula of Haiti, is about 300 kilometers long, and it's only a segment of 50 kilometers in the middle that released its energy during the earthquake.
NARRATOR: The rest of the fault remains under stress. Calais predicts that segments even closer to Port-au-Prince could snap at any time.
ERIC CALAIS: Those segments have been brought slightly closer to the next earthquake. Again, what's the timeline? We don't know.
NARRATOR: Port-au-Prince is not alone in its predicament. Anywhere on the globe above an active fault is vulnerable. And the faster the underlying plates move, the more frequent the quakes. The Middle East, South Asia, China, the Pacific Rim, in the absence of prediction, these zones remain some of the world's deadliest places.
And let's not forget California, home to the San Andreas fault. This infamous fault system stretches 800 miles, from Northern California to the Mexican border. Like the fracture under Haiti, it's a vertical "strike-slip" fault, meaning the plates grind past one another horizontally. But here the plates move more than three times faster, at over an inch a year, generating powerful earthquakes.
In the south, some sections have been quiet for over a hundred and fifty years. Now satellites measuring ground shift reveal a buildup of stress energy equivalent to 3,000 nuclear bombs. The next rupture could be the "big one." And it's the L.A. region that's under threat.
Tom Jordan of the Southern California Earthquake Center has spent years preparing for this quake.
TOM JORDAN: We have something like 800 scientists working together on a series of projects, trying to really understand the earthquakes in Southern California, where we have a very complex fault system, very active, a lot of risk to the 20 million people who live here.
This is earthquake country. We don't know when that earthquake or sequence of earthquakes will occur, but they're inevitable.
NARRATOR: The last lethal quake on the San Andreas fault system was the 1994 Northridge earthquake, which killed over 60 people and caused 20 billion dollars of damage. And that was only a magnitude 6.7.
Jordan and his team believe that levels of stress now indicate the rupture on the main fault could be 30 times greater. The city recently played out a worst-case scenario.
TOM JORDAN: We had an exercise that we developed for southern California in 2008. We call it "The Great Southern California Shake Out." We created a scenario of a very large earthquake—magnitude 7.8 earthquake—on the San Andreas fault, just east of Los Angeles, and we asked the question: what would happen if that earthquake occurred?
NARRATOR: The conclusions? Over 200 billion dollars of damage; millions of people displaced; and thousands of deaths.
ARNOLD SCHWARZENEGGER (Governor of California) If this earthquake would have happened in reality, there would have been buildings coming down. We know that there would be no water, now, in certain areas. So that's what this exercise is all about.
NARRATOR: If scientists are right, millions of people face a massive disaster.
TOM JORDAN: The San Andreas fault is very capable. You might say it's locked and loaded.
NARRATOR: With so much at stake, some seismologists are exploring new methods of forecasting when a quake will happen. Probing deep inside the hazardous faults, they are learning to listen for clues.
Anza-Borrego State Park, Southern California: This rig is drilling for something more precious than gas or oil. It's part of an ambitious attempt to find the Holy Grail of seismology: underground activity that could warn of the next major earthquake.
Geologist Frank Vernon is in charge.
FRANK VERNON (University of California, San Diego): So this is your third drill string here? Third stick, going in.
NARRATOR: They are just a few hundred feet from the San Jacinto fault, an active offshoot of the San Andreas system.
FRANK VERNON: We actually have a lot of earthquakes occurring in here, as I've said. We've had four magnitude fives in the past decade; we have magnitude fours on the average of about once a year. Four days ago, we had a magnitude 5.6, 10 kilometers over here. It's a very unique place, so this is the reason why we want to work here.
NARRATOR: The team uses the rig to plant sensors deep into the rock, embedding them 800 feet down, shielded from surface noise.
Here, as clashing plates squeeze and distort along the fault line, tiny tremors and pressure changes are produced. The sensors detect them. Any one of these signals could be the clue that a major quake is imminent.
FRANK VERNON: You're measuring the change in shape of the earth right underneath us in these bore holes. When an earthquake happens, you will see a change in that shape. There will be slight changes that we can observe.
NARRATOR: But identifying a specific tremor or pressure change that always precedes a major quake hasn't been easy.
Over the last four years, Vernon's team has surrounded the fault with eight borehole sensor stations. They've recorded many small earthquakes and are now sifting through a huge amount of data. The race is on to find a signal that is common to all of them.
FRANK VERNON: We're a long way from real earthquake predictions. It's not like we're going to achieve it today or tomorrow. It's...that's a...quite a bit further down the road.
NARRATOR: Though they haven't found it yet, this method of forecasting earthquakes holds promise for the future. But for now, the earthquakes keep on coming.
Just weeks after Haiti, another massive quake.
Chile, South America; February 27, 2010; three in the morning: This quake reaches an incredible magnitude 8.8, the fifth largest ever recorded. Daybreak reveals the scale of the damage: half a million homes damaged or destroyed and hundreds dead. The quake is so violent it accelerates the Earth's rotation, knocking a millionth of a second off the length of the day.
It is nearly five hundred times larger than the Haitian tremor. So why is this quake so powerful?
Soon
after the disaster, geologist Mike Bevis arrives in Chile to find answers.
MICHAEL BEVIS (Geophysicist, Ohio State University): This place repeatedly has huge earthquakes. Right now, the number one and the number five earthquakes of recorded, you know, history, are right here. So that makes this the world champion place for big earthquakes.
NARRATOR: All of Chile's strong quakes originate off its Pacific coast, so that's where Bevis is looking for evidence. The island of Santa Maria sits just 60 miles east of the boundary of the South American plate and the Nazca Plate. This fault, under the Pacific Ocean, triggers the enormous quake.
This beach is one of the closest points to the offshore epicenter. At the base of the cliffs, Bevis notices a white streak, a clue to the quake's sheer power.
MICHAEL BEVIS: Alright, so here we are looking at this big rock. It's in situ; it's got all of this white concretion, which is something that forms under the sea; it's got seaweed. There are actually limpets and mussels and things attached to the rock. I can see some right up there, more than four feet above my head. So, what this is telling you is that the whole coastline jumped up two to three meters.
There's two minutes of ground shaking like crazy, and during that two minutes, this whole coastline lifted up and all these marine creatures are now stranded in the air.
NARRATOR: The eight foot of uplift happened because the plates here are not grinding past one another side by side, like the faults under Haiti and California. They are subducting, one pushing beneath the other.
When the fault ruptures, the overriding plate punches upward, creating an extremely powerful quake.
BILL MCGUIRE: When you are getting subduction, the overriding plate has actually been caught on the plate that's being pushed down, and that, over time, accumulates a massive amount of energy.
NARRATOR: The enormous areas of plate that shift and the huge uplift produced give these epic quakes their name: megathrusts. And they have a chilling side effect: tsunamis. The uplift raises the sea above, creating a wave. It travels across the ocean at over 500 miles per hour, wreaking havoc.
In 2004, a 9.3 megathrust, 150 miles offshore leads to the infamous Indian Ocean tsunami that kills almost a quarter of a million people.
In 2010, the Chile quake generates its own tsunami, engulfing coastal villages like Dichato. The wave destroys over 90 percent of the town.
Mike Bevis and geophysicist Frederick Blume survey the aftermath.
FREDERICK BLUME (University of Colorado): Oh, my goodness, there's a...it's like a child's sock, hanging up 20 feet above our heads. I think that sock is probably the high-water mark. That's easily 30 feet above sea level, easily 30 feet. My goodness, nobody stood a chance here.
NARRATOR: When the leading edge of a tsunami hits a shallow shoreline, it slows down, but as the faster water behind backs up, the wave swells.
Here in Dichato, the wave reaches the upper floors of buildings.
FREDERICK BLUME: It looks like the water took out the drywall about halfway up the second floor.
MICHAEL BEVIS: Yeah, about waist height. There's waist-height damage everywhere.
Look at this: fish on the floor, my goodness, lots of fish.
FREDERICK BLUME: Here, next to the fish, "No se ovide. Don't forget, today I will be thinking of you. Happy Valentine's Day."
Wow, I hope these people knew what to do when it happened.
NARRATOR: The wave's height explains its destructive power. A 30-foot wave exerts a pressure equivalent to the weight of a battle tank. As it flows in and out, it scours the earth, destroying foundations and undermining buildings.
MICHAEL BEVIS: Well, when I was a surfer they used to tell us that one cubic meter of water weighed about a ton. So you've got a 30-foot wave, there's an awful lot of force. It's like a wall of water that comes in and never goes away.
So the currents are just pushing and pushing and pushing for minutes in the same direction and just rip everything apart, it just doesn't stop coming.
NARRATOR: Predicting and forecasting megathrusts is critical for North America. The Aleutian fault causes many quakes along Alaska's coast, and in the 1980s seismologists realize the Cascadia fault that lies off of Oregon and Washington is also active and can generate powerful megathrusts. The cities of Portland, Seattle and Vancouver are all at risk from a quake that could strike above magnitude 8. Forecasting the likelihood of the next Cascadia quake is vital.
On land, evidence is hard to find, but offshore, the answer is being discovered, buried in the mud.
A cold storage room at Oregon State University: here, hovering a few degrees above freezing, are tubes containing clues to all the quakes that have hit this region in the past few thousand years.
They're the prized possession of Professor Chris Goldfinger.
CHRIS GOLDFINGER (Oregon State University): These are, essentially, a deep-sea library. These are core samples collected in oceanographic expeditions over the last 50 years or so. So these core samples can show us things about paleoclimate and, in my case, earthquakes.
NARRATOR: Goldfinger spent the last decade collecting these cores directly from the Cascadia subduction fault off the coast, punching deep into the seafloor and extracting columns of sediment.
But each core tells an earthquake story. They are formed from layers of sandy deposits laid down over huge periods of time. Thousands of years of history are contained in a few yards of mud.
CHRIS GOLDFINGER: They're essentially a tape recorder, just like, just as a sound tape recorder records everything that happens in a room, this, these cores record everything that happens to the seafloor over time.
NARRATOR: To help forecast the next Cascadia quake he needs to know how often they struck in the past.
Undersea earthquakes create huge landslides that coat the seafloor. They deposit distinct, identifiable sandy layers. This is a sandy layer left by a megathrust landslide.
CHRIS GOLDFINGER: You can see that at the base of this sand layer there's a very sharp base. This is one telltale sign of the type of deposition that happens just after an earthquake.
NARRATOR: But to distinguish one earthquake from another, Goldfinger must probe deeper into each sample.
CHRIS GOLDFINGER: Using a C.T. scanner really revolutionizes how we can look inside the core. Each one of them seems to have a special character, a unique fingerprint. We can compare those from one place to another and use that to trace the earthquakes along the length of the subduction zone.
NARRATOR: Before this study, only 19 earthquakes in 10,000 years were identified in this region. Based on that, the odds of a quake happening here over the next 50 years were estimated to be, at most, one in seven.
But Goldfinger's C.T. analysis of the core samples identifies over 20 previously unknown quakes. Parts of Cascadia are twice as active as previously thought. This means the chance of a megathrust isn't one in seven, it's one in three.
CHRIS GOLDFINGER: This isn't just a remote possibility; this is a likely possibility. Those numbers mean that chances are actually pretty good that it's going to happen in our lifetime. It will actually happen in the next 50 years.
NARRATOR: A megathrust quake along the Cascadia fault would also generate a deadly tsunami. In the front line are towns on the Oregon coast, like Cannon Beach. When the earthquake hits, this town will not only suffer intense shaking but the power of the deadly wave. Yumei Wang knows the challenge the community faces.
YUMEI WANG (Oregon Department of Geology): The Cascadia earthquake and tsunami will definitely occur, the question is when. Cannon Beach will be devastated.
We're going to have several minutes of strong ground shaking and then about 20 minutes before the tsunami starts rolling into town. If a tsunami was on its way right now and I was standing right here, I would die. The velocities would be tremendous; you wouldn't be able to outrun it.
The tsunami can look like a breaking wave, but rather than receding back it would just keep rushing in.
NARRATOR: The people of Cascadia face a deadly double hit. But there may be hope. One hundred miles north of Cannon Beach, scientists are finding traces of a new type of super-deep earthquake, one that may help revolutionize earthquake forecasting.
The Olympic Peninsula, Washington State: These peaceful forests hide a secret. Beneath, something is stirring. Imperceptible to human senses, a silent earthquake is taking place.
Every 14 months, tiny tremors vibrate the ground for weeks at a time. They're so quiet, no one knew they existed, but now scientists are taking a closer look, and what they see might be the future of earthquake forecasting.
KEN CREAGER: ...should be here somewhere. I see it!
NARRATOR: Ken Creager, of the University of Washington, has spent years on the trail of the silent quakes. His challenge is to find out what causes them.
For years, the earthquake tremor signals were hidden among vibrations generated by machinery, crashing waves, even the wind in the trees.
KEN CREAGER: These signals that we're seeing now, which we now know as tremor, they've been going on forever. And we've viewed them as noise in the past, because we had no idea what they were. As time goes on, we often find that, you know, one person's noise is another person's signal.
NARRATOR: To isolate the signals, Creager uses seismometers that can distinguish strong, deep tremors from weak, shallow tremors.
KEN CREAGER: These are buried just about a foot or so underground.
Make sure they're nice and quiet.
But the signals that we're trying to record are about 40 kilometers, way down there.
There we go...
NARRATOR: A stomp test helps calibrate the seismometer.
KEN CREAGER: The signals we're actually looking to try to get out of this are very much quieter than the stomping I'm doing, maybe a thousand times smaller, very, very subtle signals.
And there we go; three beautiful spikes.
NARRATOR: Now Creager's data reveals new insights into how the plates move deep down.
Most earthquakes occur around 15 miles down, where the plates are locked. But around 25 miles down there is a hotter zone. Here, the plates lock briefly. Every few years they slip, with only a slight amount of shaking.
It's called "slow slip," and it generates the silent quakes.
KEN CREAGER: ...very exciting, because it tells us something about the way plates slide past each other that we had no clue about before.
NARRATOR: Identifying slow slip tremors could be critical in signaling larger quakes. During a slow slip the chance of a major quake may double. Every time the deep plate slips, stress increases on the locked crust above. Eventually a slip could trigger a major quake. Detecting the onset of these silent quakes could provide a warning that a large quake may be imminent.
KEN CREAGER: I'm optimistic about the future. I think that, in principle, slow slip may precede big earthquakes and so it's possible that by understanding slow slip better we'll be able to predict earthquakes in the future.
NARRATOR: Slow slip research is in its infancy. For now, seismologists must rely on other factors to determine earthquake threat levels.
Earthquakes can be triggered by other quakes. Energy released by one quake can travel along a fault, causing it to unfasten like a zipper.
BILL MCGUIRE: What happens then is it transfers strain onto the next bit, and it brings forward the time that that ruptures and generates another earthquake, and then that does the same to the next bit of fault.
NARRATOR: This domino effect was recently observed near the US/Mexican border.
Mexicali, April 4, 2010: A magnitude 7.2 quake strikes close to this border town. No one is killed, but over the following months it triggers a series of smaller quakes, spreading over the border.
Could the transferred stress eventually trigger the "big one" in Southern California?
TOM JORDAN: I have a sense of foreboding right now. We're a little bit nervous, because that could be foretelling the possibility of a big rupture on one of the faults here in Southern California. You know, I think the chances of us having a large earthquake are very significant.
NARRATOR: But could earthquake threat levels ever rise globally? Could the recent Haiti, Chile and Mexico quakes all be related to a global increase in seismic activity?
BILL MCGUIRE: Over the last few years, this concept of global clustering of earthquakes has gained some ground. Some geologists have suggested that there is this global clustering with, maybe, a cycle of fifty years, and that it's related in some way to stress changes in the earth's crust, as a whole. There may be some common phenomena occurring that's causing more earthquakes.
NARRATOR: But there's no proof that global clustering was a factor in 2010. Seismic activity was within normal levels.
BILL MCGUIRE: The Haiti earthquake, magnitude seven, is not particularly rare. And we might see, sort of, several or a dozen of those, maybe, a year. The great earthquakes, like Chile, magnitude eight and above, we see about one of those a year.
NARRATOR: Twenty-ten stands out because its earthquakes killed nearly a quarter of a million people.
TOM JORDAN: When we get earthquakes that cause a lot of damage, kill a lot of people, our awareness of earthquakes rises. And that's what we're seeing now. It is nothing anomalous in terms of the seismic activity. This is the earth doing its thing; this is what we have to expect in terms of large earthquakes.
NARRATOR: The recent tragedies are a reminder that despite almost a half century of research, seismologists are no closer to predicting earthquakes. The task may be impossible.
TOM JORDAN: Thirty, forty years ago, we were very optimistic that earthquake prediction was, kind of, around the corner. We thought there was a lot of new information and new understanding of the earthquake process that would allow us to predict earthquakes. Now, in fact, there's some suggestion that we may never be able to predict earthquakes with high certainty.
NARRATOR: Without high certainty, getting people to take action is difficult.
BILL MCGUIRE: For a prediction to be useful to any degree at all, we have to be sure that the earthquake is going to happen. Fifty percent's no good. You're not going to evacuate a major city and involve massive disruption of population and industry and commerce and everything else on the idea that an earthquake has a 50 percent chance of happening. If prediction is going to be useful, it has to be 100 percent certain.
NARRATOR: But you don't always need to predict a quake to sound the alarm. Having even a few seconds to respond could save lives. And earthquakes make this possible by generating their own warning signal.
Dr Richard Allen works at the University of California at Berkeley, a college built above the Hayward fault, in Northern California.
RICHARD ALLEN (Seismologist, University of California, Berkeley): This is an expansion joint in the stadium, and you can see that it's opened up, as a result of the continual motion on the fault. It comes right beneath the stand, continues across the field, beneath the goalposts and on across campus.
NARRATOR: The Hayward fault runs parallel to the San Andreas, on the other side of the San Francisco Bay. Berkeley's location makes it a perfect place for ShakeAlert, America's first earthquake early warning system.
Earthquakes generate different types of seismic waves, radiating out faster than speeding bullets. Travelling at over 15,000 miles per hour, the fastest are the primary, or P-waves, but they rarely cause more than a jolt. They are followed by the slower S-waves and surface waves. These slower waves generate the large ripples in the ground and cause most of the damage.
RICHARD ALLEN: That's where this crucial warning time comes from; the difference between the P-wave and the S-wave. In a rupture on the San Andreas fault, the P-wave would come rushing across the Bay Area at about seven kilometers per second. We can detect it; we can issue a warning before the much slower S-wave gradually comes across and does all of the damage.
NARRATOR: Providing adequate warning is a race against time. The P-wave must be detected and the alert sounded in a split second before the destructive S waves follow.
The tools for the job are kept underground or buried deep in hillside bunkers.
RICHARD ALLEN: This was built after the Second World War to monitor nuclear tests. Now we use it for our research.
NARRATOR: These are the finest Swiss-made seismometers. Their precision allows them to detect very weak tremors, locating earthquakes and estimating their magnitude with extreme accuracy.
Allen and his team have connected over 400 seismometers in a huge network across California. Buried beneath the soil, they wait for the next quake, ready to sound the alarm the moment a P-wave is detected.
RICHARD ALLEN: Each of these stations is sampling the ground shaking at 100 samples per second. It's huge volumes of data. And by having very fast computers and very efficient new algorithms, we can process it all simultaneously, within just a fraction of a second.
NARRATOR: The speed of an electronic warning signal is much faster than the slower S-waves, providing critical seconds to prepare. P-wave warning systems already operate in high earthquake-risk countries like Japan. When detected, trains automatically halt, gas mains seal, and alerts sound in many schools, businesses and homes.
With ShakeAlert in place, Californians will be able to take similar evasive action.
RICHARD ALLEN: I do not want to be surprised by the next big earthquake. Instead of being surprised by sudden shaking, I want my phone to start beeping at me, so I can get underneath the table. And I think that's very reasonable to see that happening in, here in California, in the next five years or so.
NARRATOR: But there are limits. If a quake is hundreds of miles away, ShakeAlert could provide up to two minutes warning, but the closer it is, the less warning is possible.
BILL MCGUIRE: P-wave warning systems help, but they are not the answer.
If you are right on top of the earthquake epicenter, if it is happening right underneath you, then P-wave detectors are of no use. The earthquake will hit you before they can do any good at all.
NARRATOR: Many scientists now believe the real answer to the earthquake threat lies in engineering, not geology. In the January 2010 quake, the vast majority of Haitians who died were killed by collapsing buildings.
BILL MCGUIRE: As most seismologists will tell you, it's buildings that kill people, not earthquakes. If we constructed properly in most areas of high seismic hazard, then the death tolls would drop dramatically.
NARRATOR: The challenge for engineers is to design buildings that can survive destructive vertical and horizontal shaking. Engineers now use enormous shake tables to test new designs. Their huge hydraulic pistons mimic powerful tremors. Results are helping revolutionize building designs.
Damaging Earthquake motion is minimized using deformable or floating foundations or offset by complex counterweights. Skyscrapers are built with confidence, in some of the most earthquake prone regions on the planet.
In the U.S., and in most developed countries, construction in earthquake zones is now controlled by building codes. But in places like the Pacific Northwest coast, the engineering challenge is greater. Buildings here must also deal with tsunamis.
BILL MCGUIRE: Right down that coastline, there is a problem with big waves coming in after this earthquake, and I'm not convinced that the communities there are prepared for that at all.
NARRATOR: These images are from the 2004 Indian Ocean tsunami. There is nowhere to run. The fast-flowing debris destroys one building after another.
Residents along the Cascadia fault in the Pacific Northwest face the same fate. But one Oregon town is trying to change all that. The residents of Cannon Beach have a unique plan to keep their heads above water. They are planning an extraordinary new City Hall.
YUMEI WANG: What we're looking to do is to tear down this building and replace it with a new building that is both earthquake- and tsunami-resistant.
NARRATOR: This design is called a Tsunami Vertical Evacuation Refuge: a survival platform, over 30 feet high, with space for over a thousand people, most of the population.
Extensive testing recreates the impact of intense shaking: waves, debris and scouring from the tsunami, ensuring the structure can withstand punishment.
YUMEI WANG: It can take very large forces. Not just water with sand, but also parts of buildings and cars coming and slamming into the building.
NARRATOR: Tensioned steel cables hold it together and re-center it after the earthquake. Reinforced concrete pillars allow water and debris to flow through or minimize impact with their rounded profiles. And extra deep foundations keep it standing, as surrounding soil washes away.
YUMEI WANG: This building will remain intact. It would be, possibly, the only building left standing in this whole neighborhood.
What we have in mind is, ideally, to have dozens of these, up and down the coastline, so that all of the low-lying communities with tsunami hazards have these to protect their people.
NARRATOR: These revolutionary designs may transform many buildings along the coast from deathtraps to lifesavers.
Over the last quarter century, seismologists have made huge strides in understanding how and why earthquakes happen and calculating where, if not when, they will strike. The disasters of 2010 prove that prediction remains tough, but the knowledge engineers now gather can make us safer.
BILL MCGUIRE: I don't think we can ever expect to conquer earthquakes, in the sense that we can predict them to the day and we can cope with them so that no lives are lost and no buildings fall down. We have to live with them; they are a natural phenomena. They've occurred through our earth history; they're going to continue to occur.
TOM JORDAN: What is crucial is that we be able to live with earthquakes and to sustain earthquake damage in a way that we can bounce back. We're looking to develop resilient communities, communities that can deal with these facts in a smart way. We want to keep disasters from turning into catastrophes.
NARRATOR: Today, surviving an earthquake comes down to how well you prepare for the worst. Until seismologists make a breakthrough in prediction, communities must be ready for the unexpected, because the next quake could strike at any time.
Broadcast Credits
Deadliest Earthquakes
- Written and Directed by
- Peter Chinn
- Edited by
- David Dillon
- Series Producer
- Yavar Abbas
- Camera
- Paul Lang
Chris Sutcliffe - Sound Recordist(s)
- Paul Gunn
Paul Miller - Narrated by
- Craig Sechler
- Music
- Justin Nicholls
- Animation
- Weave
- Production Manager
- Nikki Tilley
- Production Assistants
- Rosie Colman
Louise Troen - PRODUCTION CO-ORDINATOR
- Scarlet Mehta
- Online Editor
- Adriano De Mello
- Audio Mix
- Matt Faulkner
- Research
- Katie Brimblecombe
- Archival Material
- America by Air
AP Archive
Artbeats
CNN Image Source
CSI Ministries and H.O.P.E. Orphanage
Discovery Communications, Inc
E-Defense (National Research Institute for Earth Science and Disaster Prevention, NIED)
Geoeye Satellite Image
ITN Source
NOAA Ocean Media Center
O.H. Hinsdale Wave Research Laboratory, Oregon State University
Peggy Hellweg, Berkeley Seismological Laboratory
Photolibrary (OSF)
TerraTV Chile
Thought Equity Motion
TV Azteca
Globo Comunicações e Participações S.A
TVN
U.S. Geological Survey
Washington State Department of Transportation
Water Street Releasing - Special Thanks
- Duncan Agnew, University of California
Ramon Arrowsmith, Arizona State University
Brian Atwater, U.S. Geological Survey
Roger Bilham, University of Colorado
Bodo Bookhagen, UC Santa Barbara
Ian Buckle, University of Nevada
Marco Cisternas, Pontificia Universidad Catolica de Valparaiso
Robert A.Dalrymple, Johns Hopkins University
David E. Haddad, Arizona State University
Lisa Grant Ludwig, University of California, Irvine
Mike Mahoney, FEMA
Timothy Melbourne, Central Washington University
David Oppenheimer, U.S. Geological Survey
John W. van de Lindt, University of Alabama - FOR PIONEER PRODUCTIONS
- Executive Producer
- Stuart Carter
- Head of Development
- Jeremy Dear
- Head of Production
- Kirstie McLure
- Head of Finance
- Peter Dunkerley
- NOVA Series Graphics
- yU + co.
- NOVA Theme Music
- Walter Werzowa
John Luker
Musikvergnuegen, Inc. - Additional NOVA Theme Music
- Ray Loring
Rob Morsberger - Post Production Online Editor
- Mike Amundson
- Closed Captioning
- The Caption Center
- Publicity
- Eileen Campion
Victoria Louie
Karen Laverty - Marketing
- Steve Sears
- Researcher
- Kate Becker
- Production Coordinator
- Linda Callahan
- Paralegal
- Sarah Erlandson
- Talent Relations
- Scott Kardel, Esq.
Janice Flood - Legal Counsel
- Susan Rosen
- Post Production Assistant
- Darcy Forlenza
- Associate Producer Post Production
- Patrick Carey
- Post Production Supervisor
- Regina O'Toole
- Post Production Editors
- Rebecca Nieto
- Post Production Manager
- Nathan Gunner
- Compliance Manager
- Linzy Emery
- DEVELOPMENT PRODUCER
- Pamela Rosenstein
- Supervising Producer
- Stephen Sweigart
- Business and Production Manager
- Jonathan Loewald
- Senior Producer and Project Director, Margret & Hans Rey / Curious George Producer
- Lisa Mirowitz
- Coordinating Producer
- Laurie Cahalane
- Senior Science Editor
- Evan Hadingham
- Senior Series Producer
- Melanie Wallace
- EXECUTIVE PRODUCER
- Howard Swartz
- Managing Director
- Alan Ritsko
- Senior Executive Producer
- Paula S. Apsell
A NOVA Production by Pioneer Productions for WGBH
© 2011 WGBH Educational Foundation
All rights reserved
Image:
- Image credit: (Haiti after earthquake)
- © Claudia Dewald/iStockphoto
Participants
- Richard Allen
- Seismologist, UC Berkeley
- Michael Bevis
- Geophysicist, Ohio State University
- Eric Calais
- Seismologist, Purdue University
- Ken Creager
- Seismologist, University of Washington
- Chris Goldfinger
- Oregon State University
- Tom Jordan
- Director, SCEC
- Bill McGuire
- University College London
- Frank Vernon
- Seismologist, UC San Diego
- Yumei Wang
- Engineer, Oregon Department of Geology
Preview | 00:30
Full Program | 53:05
Full program available for streaming through
Watch Online
Full program available
Soon