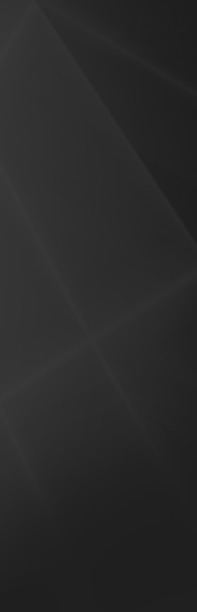
Making Stuff Safer
Host David Pogue examines groundbreaking research that aims to keep us out of harm's way. Airing November 6, 2013 at 9 pm on PBS Aired November 6, 2013 on PBS

Program Description
Transcript
Making Stuff Safer
PBS Airdate: November 6, 2013
DAVID POGUE: Civilization is built on the human drive to invent. We take the raw stuff of our planet, the materials that give names to the ages—stone, bronze, iron and more—and craft them into new forms, expanding our horizons, exploring hidden worlds and engineering life-changing technologies, always pushing the limits to be colder, faster, safer, wilder. And now a new era is upon us. I'mâ¦
Fire! Fire!
â¦and I'm on a quest to live on the edge and tame our dangerous world. But it's not easy! Think of what Mother Nature throws at us. Is there a way to meet her threats with new technologiesâ¦
Wow, in about two seconds that thing was out!
â¦including buildings that can stand up to a shakedown?
JOHN VAN DE LINDT (Colorado State University): Wow!
DAVID POGUE: And what about threats that seem totally unnatural?
This is uranium?
Can we design defenses to thwart the bad guysâ¦
MICHAEL SOSSONG (Decision Sciences International Corporation): There's something high density in there.
DAVID POGUE: â¦and protect innocent lives?
So this plant can sniff bombs now?
Can engineers protect us from ourselves, with cars that can read the road?
It's not slowing down very much at the curve!
COACH: (Keep your head up! Keep your head up!)
DAVID POGUE: Can we make better gear for our kids?
EVAN BREEDLOVE (Purdue University): That allowed us to see how hard the hits are.
TOM TALAVAGE (Purdue University): We're here to make the game safer.
DAVID POGUE: We'll never live in a risk-free world. And who'd want to?
Are you saying that human trials are beginning right now?
PAULA HAMMOND (Massachusetts Institute of Technology): Here we go!
DAVID POGUE: But we might be able to breathe a bit easierâ¦
Whoa, that was fast!
â¦by Making Stuff Safer.
In Northern California, Stanford University professor Chris Gerdes is hard at work in his favorite lab—the race track—trying to make cars safer.
I hear you are messing around with automotive safety issues.
J. CHRISTIAN GERDES (Stanford University): Trying some new ideas.
DAVID POGUE: Sorry, I couldn't hear you; a racing car just went by.
It looks like he's enlisted the help of an unflappable driver, who never flinches in the face of danger. He's really pushing that car to its limits! I'm dying to meet this intrepid maniac, who seems willing to do anything in the name of science.
Hey, that was reallyâ¦hey, come on! There is no driver! What have you done? What kind of satanic ritual is this?
CHRIS GERDES: This is Shelley, our self-driving Audi TTS.
DAVID POGUE: Self-driving racing car? That's right. There's no one at the wheel! Shelley drives herself. And even though she's got a lead foot and a sweet tooth for squealing tires, her whole purpose in life, or in silicon, anyway, is to keep human drivers alive. It may sound crazy, but Chris designed this robotic speed demon in order to make our cars safer. After all, we take a calculated risk every time we get behind the wheel. Look at all the terrible drivers out there.
So is there an alternative? What if our cars could drive themselves? That brings us back to Shelley. Turns out, she's not just a piece of amazing engineering. She actually helps Chris study how to push a car to the very limits of physical performance without losing control.
CHRIS GERDES: One of the things that's inspired us is to look at how the very best drivers control the car. So we've looked at racecar drivers who are able to get every bit of the friction between the tire and the road.
DAVID POGUE: Many accidents occur when tires lose their grip, when the forces needed to keep the car on the road overwhelm the force of friction. That can put the car into a spin or make it impossible to steer.
Racecar drivers are masters of the road. They push their cars to the limit, at the very edge of control.
CHRIS GERDES: In racing, you get to the limits of the car's capabilities, because you're trying to be fast. In ordinary driving, you may get to the limits of the car's capabilities simply because you're on an icy road. The exact same physics apply, in these two cases.
DAVID POGUE: So Chris wondered, what if he could transfer the reflexes of a racecar driver into the operating system of a regular car? That would be like giving the ordinary driver a computer co-pilot to take over whenever the car begins to lose its grip. But how would he do that?
His first step was to wire high-performance cars with sensors and have racecar drivers speed around the track, as fast as possible, right up to the point of skidding out of control. The sensors recorded how the pros steer, accelerate and brake.
Then Chris translated that driving prowess into software for Shelley's on-board computer. Her two G.P.S. antennas, accelerometers, gyroscopes and other sensors feed her computer real-time data about position and speed. The computer then sends commands to the steering system, throttle and brakes.
Okay, seeing is believing. Unfortunately, Chris seems to think riding is believing, and he wants me to put my life in the hands of Shelley's computer.
CHRIS GERDES: We're off.
DAVID POGUE: Just like a big expensive toy.
Okay so you'reâ¦
CHRIS GERDES: I'm not doing anything right now.
DAVID POGUE: This is not slowing down very much at the curve!
CHRIS GERDES: There's no need to.
DAVID POGUE: It just drove off the road, dude!
CHRIS GERDES: If you don't go up on the rumble strip then you're slow. Any racecar driver will tell you that.
DAVID POGUE: What a smart little car.
Shelley is constantly adjusting her position on the track. Her adjustments just aren't very smooth! But I guess smooth doesn't matter if your only priority is staying on the road. Fortunately, Chris can take over at any time.
But understanding how the best drivers drive is only part of the equation. Chris needs to know more about how ordinary, accident-prone drivers perform, in order to figure out how a computer might help them out. So he and his team have developed another car.
CHRIS GERDES: Welcome to X1. This is an entirely student-built research vehicle that we're using to develop some of the algorithms for Shelley.
DAVID POGUE: It's cool. It's kind of like a dune buggy effect.
Researchers can test all kinds of driving conditions with the X1, by controlling each wheel, individually, to simulate a change in friction between the tire and the road.
CHRIS GERDES: The rear wheels actually steer out, causing the car to go into a spin, and we watch the reaction of the driver to correct that.
DAVID POGUE: All right, so you're going to take it for a drive?
CHRIS GERDES: No, David, we we'd really like to get your data. So we'd like to wire you up and get you to drive the car.
DAVID POGUE: Oh, so the test subject is me? Shocker!
LENE HARBOTT (Stanford University): I'm going to put some E.E.G. electrodes on your scalp. We can monitor your brain activity, as you drive X1 around the skid pad.
DAVID POGUE: I don't know, I think my brain activity might blow out your equipment.
These electrodes won't reveal what I'm thinking, but they'll record patterns in my brain activity, an indication of how hard my brain is working.
I'll be back.
And now I meet my co-pilot.
HOLLY RUSSELL: (Stanford University): I'll be messing with you a little bit. I designed the experiment that we're going to be doing. And while you're driving, I'll be collecting your data, here, on the computer. Are we ready?
DAVID POGUE: Whenever she wants, Holly can take control of any of the four wheels. Any moment now, she'll make the X1 behave as though we've hit a patch of ice.
The squealing is okay?
HOLLY RUSSELL: Yeah, you might want toâ¦oooh. Okay, there you go.
CHRIS GERDES: Not bad.
LENE HARBOTT: This is where Holly triggered the rear wheels to go through a very sharp angle change, to make you feel like you're starting to spin out.
DAVID POGUE: There's an abrupt change in my brainwaves, suggesting I've become super-focused on controlling the car; so focused that if this were a real life situation, I might not notice something as obvious as an oncoming bus.
This data could lead to cars that know just when to step in to bail human drivers out of hairy situations. Which reminds me, I have one final question about self-driving Shelley: how does she stack up against a real pro?
Let's find out. David Vodden has 50 years of experience and knows this track inside and out. But to set a high benchmark, I'm going to take the first lap.
DAVID VODDEN (Thunderhill Raceway Park): Good exit. Good line.
DAVID POGUE: Come on, baby, come on. Give me some horses!
CHRIS GERDES: Two minutes, fifty-one seconds. You've set the mark for this race, and we'll see how the others do.
DAVID POGUE: Woohoo! Two-fifty-one! I'd like to see a piece of software beat that!
The time to beat is two fifty-one, old man.
DAVID VODDEN: Old man?
DAVID POGUE: A professional driver relies on experience, intuition and the feel of the road. He's always thinking ahead.
CHRIS GERDES: This is the warm-up lap.
DAVID POGUE: This is his warm-up lap?
He pushes the car to its limits and smoothly reins it back in.
CHRIS GERDES: Two-nineteen-point-three.
DAVID POGUE: Two-nineteen?
CHRIS GERDES: He's a little bit faster—by more than thirty seconds.
DAVID POGUE: 'Cause you're using a phone instead of a real clock, that's why!
Now Shelley's handlers prep her for the big moment.
PAUL: Start the model.
JOE: Model started.
PAUL: You are now able to exit the vehicle.
DAVID POGUE: Unmanned vehicle on the track!
Shelley makes 200 calculations a second to determine her correct speed and brake-point, based on the available friction.
Robotic, ghost-driven vehicle!
When our cars are this smart, we'll be a lot safer!
So who won, man or machine?
CHRIS GERDES: In third place, with a time of two-fifty-oneâ¦
DAVID POGUE: In second place, with a time of two minutes, twenty-one seconds, Shelley; and today's winner, with a time of two minutes and nineteen seconds: David Vodden.
Today, man beat machine by just two seconds. But Chris is confident that Shelley will beat even the best of us someday soon and make the cars we drive much safer. That is, if we manage to hang onto our spot behind the wheel at all.
It may seem like the stuff of a science fiction movie—just tell your car where you want to go and let it do the driving—but that day may not be far off. Google already has a fleet of self-driving cars that have logged several hundred-thousand miles on surface streets and freeways. They're already officially street-legal in California, Nevada and Florida. Someday, robotic cars may virtually eliminate traffic accidents. But for now, driving is an unavoidable risk that most of us accept, even though some 35,000 people a year will die on the road in the United States.
Flying is much safer. Too bad we have to put up with what happens before takeoff. The frequent flier in me wants to know. Isn't there a better way to combat the risk of terrorism that's quicker and less intrusive?
Now, the Department of Defense is funding a promising and unusual new explosives detection system. It's the brainchild of professor June Medford, at Colorado State University.
JUNE MEDFORD: (Colorado State University): They actually don't need wires, they don't need batteries, and they don't need electricity. And we'll take you down to the deep part of the basement to see them.
DAVID POGUE: This must be some serious ultra-top-secret tech!
JUNE MEDFORD: So, right, ready? Here they are.
DAVID POGUE: What? Behind the salad bar?
JUNE MEDFORD: No. These are our bomb detectors, the plants.
DAVID POGUE: Come on! It's a salad green!
JUNE MEDFORD: It's a plant.
DAVID POGUE: It can't move, it can't talk, it can't touch anything, or, smell anything.
JUNE MEDFORD: But it's got to breathe, just like you and I.
DAVID POGUE: And like us, plants can sense all sorts of things in their environment: light and moisture, temperature and touch, and chemicals, like nitrogen or phosphorous.
JUNE MEDFORD: They have ways to sense and respond to their environment continuously, 365 days a year, 24 hours a day.
DAVID POGUE: Yeah, but they're slow.
JUNE MEDFORD: They're not slow. Take, for instance, the Venus flytrap. It's fast enough that it can catch a fly.
DAVID POGUE: Snap! Whoa. Okay. That was fast.
June's plants can't catch flies, but they can detect traces of chemical explosives through microscopic receptors on the surface of their leaves, stems and roots.
If I could get in there with a microscope, what would these receptors look like? Are they little Velcro or hands?
JUNE MEDFORD: They're little proteins. We actually have a model of what one would look like, like, right here.
DAVID POGUE: Receptors are proteins that are shaped to bind, like a lock and key, to specific chemicals found in nature. But amazingly, some receptors on June's plants can latch on to manmade compounds.
Okay, I'm floating through the air. This is a particle of�
JUNE MEDFORD: Of bomb stuff.
DAVID POGUE: And it's wafting through the air?
JUNE MEDFORD: Wafting through the air. And you can't hide, and it comes in and binds to this.
DAVID POGUE: You've engineered this protein, this receptor, on this plant, to look for something new, a bomb material or explosive, that nature didn't, didn't breed?
JUNE MEDFORD: Absolutely.
DAVID POGUE: Are you genetically modifying that plant?
JUNE MEDFORD: Yes we are.
DAVID POGUE: June works with a group at the University of Washington that custom-designs new proteins. They tailor-made a plant receptor that binds to compounds in explosives.
Then they came up with the sequence of D.N.A., the gene that tells the plants to grow this receptor. And how does June get that D.N.A. into her plants? It's as easy as taking a bath.
JUNE MEDFORD: If you wanted to put a new gene in a plant, all you have to do is take your plants, turn them upside down in the solution, and give them a gentle swirl.
DAVID POGUE: What?
JUNE MEDFORD: Maybe some music.
DAVID POGUE: Come on, that's like a B-movie version of genetic modification. What about the, injector, syringe and the microscope andâ¦
JUNE MEDFORD: It's this.
DAVID POGUE: This solution contains bacteria that naturally infect plants. June inserted the new gene into the bacteria. Now, she leaves it up to them to do the dirty work of transferring the gene into the plant's D.N.A.
That can't work.
JUNE MEDFORD: Yes, it can. Here.
DAVID POGUE: That's like a kindergartener's science project.
JUNE MEDFORD: Here, you do it, David.
DAVID POGUE: Just by swishing it around upside down?
JUNE MEDFORD: Indeed, indeed. Think about plant sex. Slow, slow. There you go.
DAVID POGUE: Alright, I've done it. So this plant can sniff bombs now?
JUNE MEDFORD: The seed from that plant can sniff bombs.
DAVID POGUE: Oh, the seed. So this, this was the plant sex, and the offspringâ¦
JUNE MEDFORD: The offspring will sniff bombs.
DAVID POGUE: â¦will have the superpower.
The swirled plants eventually flower and produce seeds that June grows in Petri dishes. These tiny plants are bomb detectors, but how, exactly, can they tell us there's a bomb nearby? It's not like they can wag their tails and bark.
When molecules of explosives bind to the engineered receptors on the surface of the plant they trigger a powerful response.
And, what exactly, happened?
JUNE MEDFORD: Well, they detected a substance. It activated a histidine kinase signal transduction pathway that sent a high energy phosphate bond from a histidine to aspartate, leading to a conformational change in the protein, activating our degreening circuit, leading to loss of chlorophyll.
DAVID POGUE: What does that mean?
JUNE MEDFORD: The plants turned white.
DAVID POGUE: For now, it takes 48 hours for the plants' leaves to lose all their chlorophyll, the pigment that makes them green. June tracks the progress with time-lapse cameras. But she's working on reducing that time to just seconds. Fast as a Venus flytrap.
So, someday, this could be the front yard at the airport.
JUNE MEDFORD: That's right. This is the entrance to the airport. This is the entrance to the football stadium.
DAVID POGUE: Or a shopping center, theater.
JUNE MEDFORD: Theater. The entrances to any area, including the National Mall, in Washington, D.C.
DAVID POGUE: The only problem is, what happens, if somebody just happens to have planted white flowers? Wouldn't everyone be like, "Aaah! Bombs!"?
JUNE MEDFORD: The leaves are still green, David.
DAVID POGUE: Oh, it's the leaves that change color.
June has harnessed the incredible machinery of nature to create bomb detectors powered by water and sunshine. And now there's another new technology that may protect us against even scarier weapons like a dirty bomb, or an actual nuke.
Every year, about 16-million cargo containers arrive at American ports. The worry is that any one of them might contain dangerous radioactive contraband. Customs officials can scan only a small percentage of them. Like giant Geiger counters, radiation portal monitors can detect the gamma rays emitted by uranium, plutonium and other nuclear materials, but not all our ports are equipped with this gear. And besides, those detectors can easily be fooled. X-rays can spot nuclear materials, but they can be harmful to living things, like port officials.
What if there was a harmless way to see inside every container? It would be like having superpowers.
At the southern California offices of Decision Sciences, Michael Sossong is working on just such a system. But before he shows me how it works he's going to demonstrate the shortcomings of standard radiation detectors.
He has me wield a Geiger counter.
MIKE SOSSONG: The nuclear materials that we're looking for are not the only radioactive things out there. There's a lot of other things that are putting off radiation that we use in our daily lives.
DAVID POGUE: Really? Like?
MIKE SOSSONG: Like bananas.
DAVID POGUE: Bananas?
Mike tells me that bananas actually contain potassium 40—a radioactive isotope—but way too little to hurt us. Still, containers of bananas have set off alarms.
So the, the port inspectors, they'd be looking with their monitors and they'd be like, "Whoa, there's something radioactive in there;" freak out everybody. And then they're like, "Oh, it's just bananas."
MIKE SOSSONG: Right.
DAVID POGUE: Water softener salt contains even more of that potassium, and some ceramics get their bright colors from radioactive glazes.
MIKE SOSSONG: Yes, and that's a, a serious problem with the detectors that we have out there now, is that they're getting hundreds of false positives, at a busy port, a day.
DAVID POGUE: It can take hours to hand-inspect shipments to confirm a false positive, and that's time port officials could use to detect real threats. But there's an even more chilling problem.
MIKE SOSSONG: We actually call this the, the "cube of terror," the type of arrangement that a terrorist might use, trying to smuggle these materials.
DAVID POGUE: It's just three layers of lead inside a steel-lined milk crate. And this is a cube of uranium. Don't worry. This is depleted uranium, not the kind used in bombs. But it's still radioactive enough that I need to wear gloves to handle it.
Oh, man, it's very heavy!
Nuclear materials are dense. This cube weighs nearly 50 pounds.
I'm all set.
MIKE SOSSONG: All right.
DAVID POGUE: Behold: one cube of terror and no radiation to give it away.
MIKE SOSSONG: You could now drive this past any passive radiation detector, and they wouldn't detect it.
DAVID POGUE: Frank! It's just milk! Don't worry about it!
But now there may be a way to peer into the cube. It's a technology based on something from outer space: cosmic rays. When these high-energy particles hit Earth's atmosphere, they create subatomic particles called muons. Traveling at nearly the speed of light, muons rain down on our planet all the time and zip through just about anything in their way, including you, me and shipping containers.
This contraption may look like nothing more than a huge metal frame, but it can detect those muons.
You know it looks like it's nothing but a bunch of aluminum pipes.
STANTON SLOAN (Decision Sciences International Corporation): You're right, it's just aluminum tubes. There's nothing, you know, very exotic in terms of the physical machine. The, there's a lot of software, a lot of complexity in the software.
DAVID POGUE: More than 4,000 aluminum tubes make up this lower detector, and 4,000 more form the upper detector. Each tube has an electrically charged wire running through it. When a muon passes through the tube, the wire sends a signal to a bank of computers. The upper detector records the path of each muon as it enters the scanner. As the muon penetrates the container and its contents, these solid objects change its trajectory a bit—the denser the material, the greater the change. When the muon hits the lower detector, the system records how much the muon's path has changed and calculates the density of every object in the container. But can it look through lead and spot a hidden block of uranium?
This I've got to see.
MIKE SOSSONG: Okay, see that box of office paper over there? Pull that out and make a nice little spot for it.
DAVID POGUE: Michael helps me hide my evil milk crate in a shipment of innocent goods.
So here's where the magic happens. As thousands of muons stream through the detector, the computer builds up an image of the container and its contents.
MIKE SOSSONG: You can already see. There's the pallets; you can see the corner posts of the container. We get a full 3D picture of what's in the container, and that's critical, because it, it helps to address this issue of hiding things behind other, more dense objects.
DAVID POGUE: Materials used in nuclear weapons are among the densest on Earth.
Within seconds the uranium shows up.
MIKE SOSSONG: This isn't just a load of paper and kitty litter. There's something high density in there. And you can actually see the nice red arrow.
DAVID POGUE: That's amazing. So, even though I put that uranium in three half-inch-thick layers of lead and inside the steel of a container, like Superman, with X-ray vision, you are seeing that block.
MIKE SOSSONG: It's actually better than X-ray vision; it's muon-vision.
DAVID POGUE: For a next step, a full-scale scanner is being tested at Freeport, in the Bahamas. If it pays off, this technology may help protect our ports against nuclear threats.
But even the best border security won't protect us from what Mother Nature routinely throws our way! Of all our planet's upheavals, earthquakes are among the most terrifying. We can't predict them, and we sure can't stop them. But, increasingly, engineers are learning how to protect against their awesome power.
That's good news, because one of my favorite cities is also one of our most vulnerable: San Francisco. I'd always thought these skyscrapers would be the hardest hit in a big quake, but, in fact, the taller the building, the more flexible it is. Skyscrapers can sway without falling over.
What we need to worry about are thousands of buildings that went up before the 1970s. They're called "soft story" buildings, because their ground floor is an open space, usually a garage, with large doorways.
In an earthquake, that structurally soft story can't support the motion of the upper floors and it collapses.
In 1989, a magnitude 6.9 earthquake damaged hundreds of these structures in the Bay Area, and left thousands homeless. Today, throughout California's seismic zones, some 2-, to 300,000 people still live in these death traps. They're sitting ducks. The risk is so high that cities across the state are writing laws requiring that these structures be retrofitted. But how can engineers figure out exactly what will work?
Well, for starters, a five-university team built a replica of a soft story building from the 1920s. But they couldn't just sit around and wait for the earth to move. They staged their own earthquake. Then they followed that with a devastating aftershock that brought this building to its knees.
But we're getting ahead of ourselves, because before they flattened it, engineers spent a month testing ways to keep the structure standing.
It's built on the largest outdoor shake table in the world, at the University of California, San Diego. Powered by massive hydraulic pistons, this 172-ton brute can simulate the most devastating earthquakes ever recorded. It can also make a mean milkshake.
Seriously, for team leader John van de Lindt this is the project of a lifetime.
JOHN VAN DE LINDT: This is the first time a building this size, that represents a true soft-story building, has been tested.
DAVID POGUE: His team will test four engineering solutions that performed well in computer models.
JOHN VAN DE LINDT: We're looking at design and retrofit methods to try and make these structures safer for the many, many families around the United States that live in them.
DAVID POGUE: The first retrofit involves panels of a new building material called "cross laminated timber," or C.L.T. C.L.T. is made of lumber, stacked at right angles and glued together, which makes for panels that are very strong and stiff.
Two sets of panels are placed on the garage floor and anchored to the ceiling. During an earthquake, they should rock in place and help absorb energy from the motion of the upper stories. But the C.L.T. panels aren't bearing the burden alone. They're attached to steel rods that run straight down into the foundation.
JOHN VAN DE LINDT: The steel rods, they have to transfer forces from the cross-laminated timber, while it rocks, and this will dissipate the energy from the earthquake.
DAVID POGUE: Before the test, they fully wire the place: 30 cameras and 300 sensors to measure the movement and behavior of each part of the building.
Now it's time to rock and roll.
DAN RADULESCU: Loma Prieta, in 10, 9â¦
DAVID POGUE: This will be a simulation of the 1989 earthquake that took 63 lives and destroyed countless structures. But with its retrofit in place, this building's ready to rumble.
DAN RADULESCU: Three, two, one, running.
DAVID POGUE: Can just a few wooden panels really prevent collapse? The original earthquake lasted about 15 seconds. That may not sound like a long time, but if you happen to be inside a building that's about to collapse, it must feel like an eternity.
Cameras reveal the C.L.T. panels rocking in place. At last the test is over. They head inside and look for damage.
It's clear that the panels saved the day, but the rocking motion put so much stress on the steel rods that they actually stretched.
JOHN VAN DE LINDT: All in all, this test was absolutely perfect, I'd say phenomenal. If this wasn't here, the building would have collapsed.
DAVID POGUE: The researchers document every crack and break. Some repairs would be needed, but the building would still be livable.
C.L.T. panels are the simplest of the retrofits they'll test. Steel frames strengthened the openings on the ground floor and performed even better than the C.L.T. panels, allowing minimal damage to the building. When engineers added plywood reinforcement on the upper floors, the steel frames kept the structure virtually intact.
Same goes for the fourth retrofit: high-tech dampers that acted like shock absorbers on the bottom floor and kept the building standing.
All in all, four for four.
JOHN VAN DE LINDT: We tested four retrofits to this point. They've done exactly what we wanted them to. Sometimes even performed a little bit better than expected. All these retrofits are a viable option.
DAVID POGUE: That's great, but what would be the point of building a four-story structure on a shake table and then letting it stand?
So now, with all the retrofitting removed, there's one last test to run. Engineers have modeled this one in the computer, but they've never actually seen a soft story building collapse, until today.
JOHN VAN DE LINDT: So the two safe areas areâ¦is back behind the red tape there, and then you can walk around the building, and you'll see red tape over here.
DAVID POGUE: For earthquake engineers, this is an epic event.
They start out with a few gentle shakes, then they gun it, hitting it with equivalent of a 6.7-magnitude shake. They watch as the second floor juts out over the garage level by nearly a foot and a half. The engineers are shocked to see that it's still upright.
JOHN VAN DE LINDT: Wow, so 17 percent drift and it's standing. I guess that answers the question on how much drift these can take.
DAVID POGUE: What will it take to bring this baby down? They hit it once again with a large aftershock.
DAN RADULESCU: One hundred percent M.C.E. level, in 3, 2, 1, running.
JOHN VAN DE LINDT: Wow. Unreal. Wow. I don't think I've seen something like that.
DAVID POGUE: The building they worked so hard to protect is just a pile of lumber, beyond repair.
JOHN VAN DE LINDT: I do have a soft spot in my heart for the building. But I know what we've done will provide engineering and science what they need to make these types of dangerous buildings much safer for the families that live in them.
DAVID POGUE: Let's hope Californians apply those retrofitting techniques before the "Big One" hits.
Luckily, most of us don't live in earthquake country, but none of our homes are beyond the reach of a far more common threat: fire.
Over the past century, death rates from fires have dropped 90 percent in the United States, mostly thanks to smoke detectors, fireproof materials and fire codes. Still, nearly 3,000 people die in residential fires each year. And now the risk of wild fires seems to be on the rise, most likely due to higher temperatures and droughts, brought on by climate change.
We have foams and chemical retardants to knock down flames and slow the spread of fire, but many of these products are toxic to plants and animals. Short of installing sprinkler systems throughout the wilderness, can't we find a better way to combat fires?
I've come to the headquarters of EarthClean, in Minnesota to find out.
Rumor on the street is you've come up with something better than water for putting out a fire.
SCOTT BOCKLUND (TetraKO/EarthClean Corporation): This is going to put the fire out faster than anything you've seen before.
DAVID POGUE: Vanilla pudding?
This goop is called TetraKO, and it's different from plain old water in an important way.
SCOTT BOCKLUND: The unique thing about it is it actually adheres to where it's sprayed. You can spray it on a ceiling, you can spray it on a wall, and it would actually stick and stay there.
DAVID POGUE: So the, the firefighter, if it were water, would have to stand there hosing, hosing, hosing, but now can just spray the goop, and then move on to the next wall?
SCOTT BOCKLUND: Exactly.
DAVID POGUE: But won't this gel just melt away in a fire?
SCOTT BOCKLUND: I'll show you that the stick does actually burn.
DAVID POGUE: Apparently, this stuff can also keep things from going up in flames.
So, what's in there, exactly?
SCOTT BOCKLUND: Well, our main ingredient is cornstarch.
DAVID POGUE: Cornstarch?
SCOTT BOCKLUND: If you'd like to try it, you can.
DAVID POGUE: Wait, what are you doing?
SCOTT BOCKLUND: It's all natural. You can eat it.
DAVID POGUE: So how does cornstarch stop fire?
ERIC MORRISON (TetraKO/EarthClean Corporation): So, you've all made gravy. So here's my cornstarch.
DAVID POGUE: Just add a little water, apply some heat, and that simple white powder becomes white goo.
ERIC MORRISON: This will thicken up, as soon as it gets hot.
DAVID POGUE: Cornstarch consists of long chains of glucose—a form of sugar—tightly packed into tiny granules. When mixed in water and heated, those chains separate. They swell and create a net-like structure that makes the mixture thicker. That's why we use corn starch to thicken gravy, and it's why this stuff sticks to walls in a fire, instead of melting.
So, next time I'm making Thanksgiving dinner, and it bursts into flame, as typically happens with me, what should I grab to throw on it? A bucket of water or the pot of gravy?
ERIC MORRISON: Pot of gravy.
DAVID POGUE: The gel also contains polymers, long molecular chains, dissolved in water, which give it the special properties of a non-Newtonian fluid.
Most fluids are just plain Newtonian. Water is the classic example. Stir it, whisk it, shake it as much as you want and it doesn't change its thickness. Juice, honey, and most oils are also Newtonian fluids. But non-Newtonian fluids change when you apply force to them.
The best-known non-Newtonian fluid is ketchup.
ERIC MORRISON: And ketchup has this property. When it's not being squeezed, it's a solid. But if you shake it up and it starts to move around, it liquefies.
DAVID POGUE: So this is a, a facial cleanser. It's a gel, but it's pretty solid.
ERIC MORRISON: It is a solid.
DAVID POGUE: The bubbles are frozen in space. And yet, through the miracle of non-Newtonian fluids, it turns into a liquid?
ERIC MORRISON: Exactly.
This is also a solid.
DAVID POGUE: And this is hair styling gel.
ERIC MORRISON: And if we pump it, it liquefies.
DAVID POGUE: It turns into a mist.
ERIC MORRISON: Right.
DAVID POGUE: The non-Newtonian firefighting gel also has a split personality.
So the TetraKO is a gel, when it's in the fire truck, and then you're spraying it, it comes out of the nozzle, instantly turns into a liquid, so it can spray. Then it lands on the wall and then what?
ERIC MORRISON: Turns back into a solid.
DAVID POGUE: Oh, my gosh!
But fire on a little paint stick is one thing. Let's see how the corn starch does at putting out a real fire, like my little fake apartment here.
The Fire Department of White Bear Lake, Minnesota helps us stage a test.
The gel hits the burning surfaces and sticks, smothering the flames. The cornstarch thickens the gel and releases clouds of steam.
Wow. About two seconds, that thing was out, and I mean completely out.
Local fire departments are using this new firefighting gel in the field. And though it costs more than just spraying water, some think it's worth it.
Chief Zikmund, can I talk to you a sec?
NYLE ZIKMUND (Spring Lake Park-Blaine-Mounds View Fire Department): You bet, glad to.
DAVID POGUE: Have a seat.
NYLE ZIKMUND: Thanks, I'll pass.
DAVID POGUE: So, you've used this stuff in real fires, out in the real world?
NYLE ZIKMUND: Out in our community, we've had it on a half-dozen fires. The crew would hit it from the doorway, and the fire's out, and it stays out. This is some of the best technology I've seen come along in years.
DAVID POGUE: TetraKO is in beta-testing for use in wildfires. It can be sprayed on vegetation and buildings in the path of an approaching fire, up to a day in advance. And it's non-toxic, safe for the environment, wildlife and us.
We've made great strides in protecting ourselves from fires, but what about protecting ourselves from the fires that flare up in our bodies? I'm talking about infectious diseases.
Vaccines are one of our greatest weapons against these deadly enemies. They've saved millions from polio, smallpox, measles, whooping cough and dozens of other illnesses. But delivering vaccines can be a challenge. Many need to be kept cold, and sometimes that's nearly impossible. And most require an injection. Where medical supplies are lacking, needles are sometimes re-used, which can spread disease. Bottom line: millions of people are not getting the vaccinations they need.
But I hear there's a team at M.I.T. working to overcome these obstacles. Scientist Pete DeMuth is developing a vaccine patch smaller than a dime.
So, what does this patch look like? It exists?
PETER DEMUTH (Massachusetts Institute of Technology): Yeah, it exists. You can take a look at a couple right here. And these needles are actually on the order of half a millimeter or less in length.
DAVID POGUE: So that'd be like this?
PETE DEMUTH: Exactly.
DAVID POGUE: And how many are there?
PETE DEMUTH: So, this patch actually has about 100 needles, but patches are made where there can be, even, thousands of needles.
DAVID POGUE: Looks harmless until you see it under the microscope.
PETE DEMUTH: All right, and then we'll bring this into focus.
DAVID POGUE: Whoa! Evil! Looks like a medieval torture device!
The next step is to coat these needles with fine layers, or films, that contain the vaccine. But how do you layer anything on something this small? You've got to know how to work at the nano-scale, down at the molecular level, like Professor Paula Hammond.
PAULA HAMMOND: Well, this is actually where we build the films that contain the vaccine.
DAVID POGUE: Hi, how are you doing?
PAULA HAMMOND: Yes, yes, we love our friend here.
DAVID POGUE: This is it, huh?
PAULA HAMMOND: This is it.
DAVID POGUE: A robotic arm dips the microneedle patch into trays containing the vaccine's components.
You, sort of, co-opted the ancient principle of chocolate fondue.
PAULA HAMMOND: The way that we make the coating, is that we take the components that make up our vaccine; one of the components is negatively charged, and the other component is positively charged.
DAVID POGUE: The microneedle patch is negatively charged. First, it's dipped in a positively-charged component and a microscopic layer forms. Then, it's dipped in a negatively-charged component, and a new layer forms. In this way, dozens of layers can be built up on the microneedles and then embedded in the skin, like a tattoo. Over days or weeks, they dissolve and release the vaccine.
But what are these components?
PAULA HAMMOND: The positively-charged ones are biodegradable polymers, in this case, plastics. And the negatively-charged ones are D.N.A.
DAVID POGUE: Wait, so, there's a polymer, and there's D.N.A., which is genetic material. I thought you said it's a vaccine.
PAULA HAMMOND: Actually, D.N.A., in our case, is the vaccine, which is very unique but also very powerful.
DAVID POGUE: Most vaccines are created from weakened or killed microbes, or from parts of microbes. The immune system mounts an attack, even though there's no real threat. Some immune cells remember the episode. Should they ever encounter the actual microbe, they'll spring into action and destroy the invader.
A D.N.A. vaccine doesn't deliver any bits of microbe. It delivers a stretch of D.N.A. that contains a gene for a bit of microbe, a protein. This gene inserts itself into our D.N.A. Now, our own cells produce proteins that trigger an immune response.
And the D.N.A. requires no refrigeration.
PETE DEMUTH: Okay, David, are you ready?
DAVID POGUE: Dude! In what universe is that a microneedle?
PAULA HAMMOND: We don't have to deal with this old technology anymore.
DAVID POGUE: Oh, that's the before, and this is the after.
PAULA HAMMOND: That was before, yeah.
DAVID POGUE: You guys are just a laugh riot.
PAULA HAMMOND: And I'm just going to apply this to your wrist.
DAVID POGUE: So, this is human trials, starting right now?
PAULA HAMMOND: Well, let's see how it goes.
All right. And we're going to place this right here.
DAVID POGUE: Ow!
It doesn't hurt at all.
PAULA HAMMOND: Just a little pressure.
DAVID POGUE: Like you're holding up a plastic game piece to my skin.
PAULA HAMMOND: It's really nice, because these microneedles are too short to reach the nerve endings in your skin.
DAVID POGUE: And, this particular one, what nasty D.N.A. is it injecting into my body?
PAULA HAMMOND: We didn't put anything on yours. This is just a blank to give you an idea of what the experience will be like.
DAVID POGUE: Wow. I don't see blood; I don't see shredded flesh, just that little ring.
PAULA HAMMOND: That's right, exactly.
DAVID POGUE: So, not only does this eliminate the problem with keeping the vaccine cold all the way from the manufacturer, not only does it solve the problem of needles being reused or misused, but it also doesn't hurt. That, you could argue, is an advantage over huge nasty pieces of metal.
PAULA HAMMOND: It's a huge advantage: no more crying children. You have happy parents, as well.
DAVID POGUE: Although, the one downside, I'd have to say, is that you don't get the little lollipop at the end for your suffering.
PAULA HAMMOND: We would like to give you a little lollipopâ¦
DAVID POGUE: Aren't you sweet?
PAULA HAMMOND: â¦for being such a great sport.
DAVID POGUE: It's a polymer!
This radical new vaccine system is still in the testing phase. If it lives up to its promise, it could eventually save millions of lives around the world.
In our pursuit of safety, we've pushed the limits of science and technology and come up with amazing innovations, and yet, we keep on doing dangerous things, sometimes just for the thrill of it. Hey, I've even pulled a few crazy stunts in the name of science! But sometimes, we're just not aware of the real risk. Take football: it's more than a sport. It's a part of American culture and a passion for millions of fans. Part of its appeal is that it's a serious contact sport.
But over the past decade, there's been a spate of stories about veteran football players succumbing to depression and dementia. And several top players have committed suicide. Autopsies of their brains revealed that an abnormal protein had destroyed cells in areas that regulate impulse control, judgment and memory. The condition appeared to be caused by a history of multiple concussions.
A concussion occurs when a violent blow to the head or sudden acceleration, like whiplash, forcefully jars or shakes the brain. Symptoms can include an inability to think clearly, blurry vision and dizziness. But scientists still don't understand exactly what happens to the brain in a concussion.
Four years ago, neuroimaging specialist Tom Talavage and his colleagues at the Purdue Neurotrauma Group began tracking football players at local high schools, because professional players are not the only ones whose brains take a beating.
BRANDON GAWLIK: I had two concussions: one in eighth grade and one in sophomore year, so, last year.
VINCE MIRELEZ: I just felt really dizzy. I felt sick to my stomach.
ALEX JONES: But I didn't think anything of it, and I went back in and played.
DAVID POGUE: The research team wired players' helmets.
EVAN BREEDLOVE: This is our sensor package, and it fits in between the padding and this space here.
DAVID POGUE: These accelerometers record the intensity and location of each hit, as well as the motion of the player's head. A wireless connection sends information to the researchers in real time.
EVAN BREEDLOVE: This may be a hit of the forehead against the helmet.
DAVID POGUE: Later, the data is translated into a plot.
EVAN BREEDLOVE: You can imagine unzipping the helmet in the back and pulling it open and flattening it out. Blue dots represent small hits. Yellow and red dots represent big hits.
DAVID POGUE: Before the season began, the researchers compiled a baseline cognitive score for each player, measuring things like attention span, reaction time and problem solving. And a functional M.R.I. scan measured brain activity, while the player performed another set of tests. The plan was to run the same battery of tests each time a player sustained a concussion.
COACH: Are you dizzy? Sick to your stomach?
DAVID POGUE: After two weeks of practice and two weeks of games, the players had sustained plenty of hits, but no one suffered a concussion.
COACH: Everything looks good.
DAVID POGUE: So the researchers retested several players anyway, expecting little to no change from their baselines. The results stunned them.
TOM TALAVAGE: This was a player who was never diagnosed with any sort of concussion. There's no reason that an athletic trainer or a coach or any other player would have ever recommended that he be examined.
DAVID POGUE: Yet his M.R.I. showed his brain working much harder on the same tests he'd aced four weeks earlier. He also showed cognitive deficits, especially in his visual memory. No concussion, but he had sustained several hundred hits to the head.
EVAN BREEDLOVE: This looks like a player who's getting their head down, charging into the line, and takes a hit every single play.
DAVID POGUE: Those hits produced the kind of impairment the research team had assumed would only be caused by concussions. And this was just one player of several with the same impairment.
Twelve weeks after the season ended, the players were tested again. Their scores returned to normal, but the surprising, earlier results forced the scientists to rethink assumptions about head injuries.
TOM TALAVAGE: What we're only starting, now, to understand, is that the players who are getting hit a lot are actually ending up just as, if not more impaired as the players who get concussions.
DAVID POGUE: Some 3,000,000 kids, under the age of 18, play football in the United States. Could repeated blows to the head, even ones that don't qualify as concussions, affect their academic performance? And just how great is the risk of permanent damage for these kids and kids playing other contact sports?
TOM TALAVAGE: These are young kids, their brains are still developing. If we are causing some low level of damage, the body is going to be spending time repairing those injuries. So, as these kids are playing more and more football, are we going to find that their brains are becoming less and less normal?
DAVID POGUE: Tom and his colleagues hope their research will answer that question. In the meantime what can we do to make young athletes safer? If we can't get them to give up football for basket-weaving, can we at least build a better helmet?
Traditionally, football helmets have been designed to prevent skull fractures, and they do that remarkably well. But how can we protect the brain inside the skull during head-on collisions and other blows?
ERIC NAUMAN (Purdue University): Every hit, whether it's from the side or the front or the top, is also going to cause the head to rotate, either back and forth, or side to side, or even in this twisting motion, or some combination of those.
DAVID POGUE: These rotational forces can be just as devastating as direct hits. So, Eric Nauman is developing a next-generation helmet, based on a double shell design. Separated by a layer of foam, the two shells move independently. In an impact, the outer shell should absorb some of the rotational force before it reaches the brain. It will take years of testing to reveal whether this new helmet can actually protect young brains during routine play.
The message about head injuries and brain damage is also reaching the playing field.
COACH: Head up! Head up! Head up!
DAVID POGUE: Some coaches have begun to emphasize proper tackling techniques, where players lead with the shoulders and get their heads out of the way.
TOM TALAVAGE: We certainly see that players who take fewer hits to the head or fewer large hits to the head tend to show fewer changes in their neurocognitive testing, in their neuroimaging. With better monitoring, better equipment and better technique, we're here to make the game safer.
DAVID POGUE: When it comes to safety, most of us think of children first and foremost. They're our future; who could blame us for trying to protect them from every peril? If we could, we'd probably dress them in bubble wrap and childproof the entire world.
But I wonder: is there such a thing as too safe? Don't we all need to test our physical limits? Isn't that how we gain the confidence we need to explore, to invent and create the innovations that improve our world? Nothing ventured, nothing gained!
Maybe the key to living well is finding the sweet spot between an acceptable level of risk and making stuff safer!
Broadcast Credits
Jill Shinefield
Joseph Kleinman
Minna Kane
Olga Arana
Peter Bonilla
Dustin Eddo
Alexandra Gallo
Chris Kellett
Ken King
Drew Levinson
Dean Miller
Patrick Schaefer
John Toffolo
Warren Wolfe
Brian Funck
Kevin Purcell
Cindy Love
Corbis
Department of Defense/US Customs
Framepool
Getty
Oddball Film + Video
T3Media
The WPA Film Library
UWTV
VA Boston/ Boston University
Robert Beckley
Defense Threat Reduction Agency
Department of Homeland Security Science and Technology Directorate
Kevin Tod Haug
Institute for Protein Design, University of Washington
Ioana Patringenaru
Dan Radulescu
Jim Schenke
Smith Memorial Playground & Playhouse
John Verrico
Tony DeRose
Dale Dougherty
Alex King
Tim McGee
Amy Moll
Mike Petrich
Ainissa Ramirez
Thea Sahr
Eric Siegel
Stephen Streiffer
Desney Tan
Jessika Trancik
Thomas Tretter
Phil Weilerstein
John Luker
Musikvergnuegen, Inc.
Rob Morsberger
Eddie Ward
Janice Flood
A NOVA Production by Gemini Productions LLC for WGBH/Boston
© 2013 WGBH Educational Foundation
All rights reserved
This program was produced by WGBH, which is solely responsible for its content.
Image:
- Image credit: (David Pogue)
- Courtesy David Pogue
Participants
- Scott Bocklund
- TetraKO/EarthClean Corp.
- Evan Breedlove
- Purdue University
- Pete DeMuth
- Chris Gerdes
- Stanford University
- J. Christian Gerdes
- Stanford University
- Paula Hammond
- Lene Harbott
- Stanford University
- June Medford
- Colorado State University
- Eric Nauman
- Purdue University
- Holly Russell
- Stanford University
- Stanton Sloane
- Decision Sciences
- Michael Sossong
- Decision Sciences
- Tom Talavage
- John Van De Lindt
- Colorado State University
- David Vodden
- Thunderhill Raceway
- Nyle Zikmund
- SBM Fire Department
Preview | 00:30
Full Program
Full program available for streaming through
Watch Online
Full program available
Soon