 |
 |
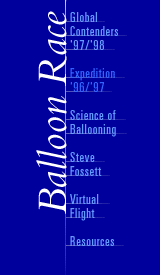
|
Interview with Leon Eversfield, Special Projects Engineer
NOVA: Leon, can you tell us exactly what you do on this project?
EVERSFIELD: I'm technically known as the Special Projects Engineer for
the Global Challenger.
NOVA: I've heard you talk about universal laws and in particular the
gas law which takes into account pressure, volume, and temperature. How does
this law apply to global ballooning?
|
Hear Eversfield
via RealAudio: 14.4 |
28.8 |
ISDN
Get RealAudio software |
EVERSFIELD: Right. There's a universal gas law, which says that
pressure times forty and times the temperature—the absolute temperature—which we measure in kelvin, degrees kelvin, that is actually a constant for any
particular gas. And that really is the principal on which the whole balloon is
flying. So this envelope which is about twelve and a half tons in weight with
the payload, has to lift that mass. So at launch, we need roughly one kilogram
of lift, is one meter cubed, or one cubic meter to lift one kilogram. So it's
a lift of twelve and a half tons. We need twelve thousand, five hundred meters
cubed of helium at sea level at about fifteen degrees centigrade.
If we just had an envelope of that volume, the moment it starts to lift, the
pressure inside that envelope would increase and we'd end up with a super
pressure balloon. And that would induce high stresses into the fabric and
potentially burst. So this balloon is a zero pressure balloon. So we actually
made the pressure larger than the twelve and a half thousand meters cubed we
need; in fact, we have a volume of thirty-one thousand, one hundred, fifty
meters cubed. And that allows the gas at sea level—we have a roughly forty
percent fill at launch, and as the balloon rises, the air around the balloon as
we go up in altitude, gets thinner and extends so the gas inside the balloon
expands.
As that expands, it gradually fills the envelope. The temperature is,
obviously decreasing as we decrease with altitude. And our target altitude is
about forty thousand feet, where it's about minus forty degrees centigrade. So
when we do the calculations, you can see that with the pressure, volume and
temperature that the balloon would actually float at equilibrium at thirty
thousand feet. With twelve and a half tons at thirty one thousand, one
hundred, fifty meters cubed.
Now, at launch, in order to get that movement upwards, we need this free lift.
And on average we have about ten percent free lift at launch. So that will be
about a ton and a half of extra force, if you like, trying to lift us up into
the air. With that free lift, we will gradually start accelerating upwards and
we will have ascended to our thirty thousand feet. The gas will have expanded
then, fully, to occupy the complete volume of the sphere.
Now, once it reaches that point, we have excess lift, if you like, we still
have an excess lift and it has to get out of the balloon. Otherwise, it starts
to over-pressurize. So we have three appendices, which are, effectively,
overflow pipes, if you like, in terms of some sort of analogy. But the helium
then goes through these pipes and overspills. And that gets rid of the excess
helium pressure inside the envelope. Now, if that cannot escape quickly
enough, the pressure will, in fact, keep rising. It's like having a hole in a
pipe—the smaller the hole, the harder it is to blow through the volumetric
flow-rate that you would require to get that excess volume out. So the three
appendices we have have been calculated that that will diminish the pressure
sufficiently quickly enough, once it reaches its float altitude.
NOVA: So the pilots will be constantly concerned about what their
altitude is and whether they're rising or descending, right? Of course at
launch they want zero wind conditions, but at altitude they are at the whims of
the jet stream.
|
Hear Eversfield
via RealAudio: 14.4 |
28.8 |
ISDN
|
EVERSFIELD: At launch, when we take off, we want zero wind conditions.
The problem is, the chances of getting zero wind conditions for launch is
fairly, fairly rare, so we need to take the opportunity when it arises.
Consequently, the jet streams may not be ideal overhead at the time. So this
may mean we wish to loiter, let's say, ten thousand feet, or fifteen thousand
feet for one or two days, which will at least give the pilots a chance to
acclimatize and get used to the new environment.
So we can ballast the balloon off at that stage to hold them at a
pre-determined float altitude below the ideal thirty thousand that they wish to
achieve. And, let's say, after a couple of days there, there's a strong jet
stream ahead at normally thirty thousand feet, they can dump some ballast and
gradually, the envelope will rise and take them to that altitude. They will
float to that altitude to whichever they've ballasted out to, and during the
day, that should remain fairly constant. As the sun comes out it will heat the
gas and the pressure temperature law comes into its own and we will gradually
rise a bit. And as the night time approaches, the temperature will drop and
then gas will contract and the balloon will lose altitude. Now, sometimes the
jet stream can be as thin as two to three thousand feet thick. So to remain
inside that jet stream, we need to heat the gas in the evening so that we can
maintain that float altitude—that ideal float altitude right in the heart of
that jet stream so that we don't fall out of it. Because, obviously, the jet
streams are carrying us on the course that we wish.
Now, also the jet stream may be taking us off on the wrong course. So we may
need to change course and the only way we can steer this balloon is by
vertically ascending or descending. That's the only real means of direction or
control of the balloon, unlike an aircraft where you can apply weather to
change direction. So in order to do that, we would need to valve helium to be
able to descend out of one jet stream into another. The danger, obviously, in
valving gas when you're at that sort of altitude, it's very, very cold,
somewhere down around the minus forty, minus fifty degrees centigrade, there's
always the small chance of ice forming. And if the valve was to be iced open,
for example, that could be potentially a dangerous situation to be in because
the valve wouldn't close and we could be losing helium, even at a slow rate,
quite constantly.
NOVA: How do you stop the valve from icing?
EVERSFIELD: We don't. We have a single valve in this balloon. There
was a big debate whether we should have two or three valves but if you've got
three valves, you've got three more chances of a valve going wrong. So this
valve has been designed and built and tested right the way down to minus sixty
degrees centigrade in a chamber that was sprayed with water to simulate more
extreme conditions than we'll ever actually see. And in fact, John Ackroyd
actually took it down to the test chambers to actually perform that series of
tests, and that was found to be extremely successful. And it's actually
powered by a BAC-111 Trim Tab Control motor which has unknown failures—It
doesn't have any known failures, and so it's an extremely reliable system.
NOVA: How do you solve your leakage problem with helium?
EVERSFIELD: The leakage problem is—first of all, you need to know
what your leakage rate is through your base fabric. So there's a lot of design
time and effort put into making sure that the base material is helium-tight.
The biggest leak path tends to come from any joints. Because obviously, the
fabric is not big enough to make in one big sphere. So it has to be split into
orange peel-type segments called gores. And we can either stitch and tape
these together—the stitching giving it the strength, the tape giving it the
sealing characteristics, we could glue it, or we could weld it. There are
several ways of welding it. We can use RF welding, radiofrequency welding. We
can use impulse welding, which is rather like a hot iron. And we can hot air
weld, which is the technique we actually finally adopted after a tremendous
test program that we went through.
Now, like any process, it's not just a matter of jumping onto a machine and
operating it. All our operators in the welding process went through a test
whereby they welded up a small test balloon and this was pressure tested and
the pressure at which it burst was recorded. And not until they'd reached the
level we'd required for structure integrity, were they then allowed to go on to
actually manufacturing the Global Balloon. Because, obviously, we need the
strength in the balloon at the welds, and we also need the correct helium
porosity.
Now, in order to ascertain what that helium porosity was on the seams, we had a
machine specifically designed for us using mass spectrometry and, essentially,
it is a machine which tests one-meter lengths at a time, you can put a seam in
of the material. It has an upper chamber and a lower chamber. The upper
chamber passes air over the top of it. It clamps it together and seals the top
and bottom sides. Air passes over the top and it checks to make sure there
isn't what we call a gross leak. For example, if you put a pin hole in it, the
air would rush through that pin hole quite quickly and you'd know you'd have a
gross leak.
If it passed that test, helium, being such a small molecule, being able to get
though much smaller holes, would then be shot across the top of this chamber
and sucked, if you like, to the underside of the chamber. And any helium
migrating from the top chamber to the bottom chamber would then be recorded by
the mass spectrometer. That was calibrated against known leaks. In other
words, if we put a pin hole in, we knew what helium leak that would give us.
The budget for the whole balloon was three hundred kilograms loss of lift for
the three weeks. We can afford to lose three hundred kilograms in the balloon
over the three weeks. Now, the base material, without any welds in it,
assuming everything was perfect, would lose one hundred kilograms in the three
weeks. So we had to ensure that the seams, of which there's approximately two
and three—three miles, minimum of seams to be welded, that those seams were,
in fact, helium tight. That allowed us two hundred kilograms of loss of helium
through those seams. So from that figure, by calculating the length of the
seams that were welded, the two hundred kilograms loss of lift in the three
weeks, we could work out what our helium leakage was for every seam.
So as the envelope was welded, we would then put it through our helium leak
detector and record every single meter of the seam that was welded to find out
what its leak rate was. That would be added up and, if necessary, remedial
action could be taken, i.e. repairs or whatever, to ensure that the helium leak
was maintained at the level which we could accept.
NOVA: What are the fabrics that will be used?
EVERSFIELD: We have three types of fabric on this particular balloon.
The upper cap is made from a polyester fabric with polyurethane coating on
either side and it is extremely tear-resistant. If I can just give you an
analogy here, you can have a very, very strong material in tension, but it can
be very weak in tear. A standard metal Coca Cola can, for example, a metal
Coca Cola can, has equal strength in tension as our fabric. So that both have
exactly the same strength. However, if you just put a small tear in the Coke
can, you can easily tear it. So although it's very strong, it tears very
easily. Our cap material, you cannot tear by hand. So we have a far more tear
resistant structure. And that's very important for something like this. So
that's the top cap, which is effectively the top hemisphere of the balloon.
That sees the highest pressure.
If you imagine filling a football with water and holding it at the top, the
bottom of the ball would have the highest pressure. And the top of the ball,
although the bottom of the ball, although there's water in it, there's very
little pressure, acting due to gravity. So the top cap carries the highest
stress.
We then have a lower hemisphere, which we've referred to as the bladder, which
is the part that has the lowest stress in it. This is actually made from a rip
stop type of hot air balloon fabric, which also has a very thin polyurethane
coating on it. And that has actually been stitched and welded with a tape—welded across the top of it to seal it, as opposed to the upper cap material,
which has just been purely welded.
And what we had to do with the top cap was to seal it with a small bead of
contact adhesive to actually stop any helium leaks progressing through the
edges of the fabric. And then, the lower cone section which leads roughly from
below the equator of the balloon down to the flying wires on top of the
capsule, that's actually a standard hot air fabric, which contains the heat
within the envelope. So those are three main materials used for the balloon.
And that is—the hot air cone is also just purely stitched, exactly as you
would do with a standard hot air balloon.
NOVA: What do you mean by welding fabric?
EVERSFIELD: The fabric, which has—can either be a nylon-based fabric
or a polyester-based fabric, is essentially just like your shirt or blouse
material. And then on both sides of that, we coat it with a thin coat of
polyurethane, which is a thermoplastic material which, when you heat it,
liquifies, and when you cool it, it resets again.
So, because the whole fabric is coated with that, that acts not only as the
helium barrier, but also allows us—when we put the two components—one piece
of fabric over the top of another one, we actually heat in between it with hot
air to a specified temperature, which melts the polyurethane and, just
immediately behind that jet of hot air running in to weld it, there's a roller
which just supplies a little bit of pressure to squeeze the two materials
together and it also cools it on its way out. So over the space of about six
inches, the whole welding process is performed.
NOVA: So, in essence, you think that the welded seams could be stronger
than the fabric itself?
EVERSFIELD: The welded seams have to be stronger than the fabric
itself. That's the usual design process. However, we have an extremely strong
fabric here so, whereas the ideal is to design it so that the failure would
never be in the joint, whether it's a glued joint or whether it's a welded
joint. That's because we have such a very strong material, we would need quite
a large overlap of weld in order to get the full strength in. But at room
temperature, yes, the weld is comparable to the strength of the material.
We've taken our temperature testing regime to all the extremes we could
possibly encounter. That's down to minus sixty, we've tested. We've tested at
room temperature. We've tested up to plus one hundred degrees centigrade,
which is the temperature of boiling water. And although it's very unlikely,
for example, the top cap will never see that temperature, it gives us a good
quality control process by which, if there is a problem, it highlights it much
earlier on and much easier to detect that you can detect at room temperature.
So those are some of the factors of safety we've built into this particular
balloon design.
NOVA: What amount of propane will you need for life support, and how do
you calculate that without actually doing it?
EVERSFIELD: Well, with the Pacific and Atlantic crossings where they've
done before—they've flown on continuous engine running all the time. At
Oswestry in Shropshire, we had the engine running for virtually five hundred
hours simulated continuous run. So that's already been done. The five hundred
hours is equivalent to three weeks. So we do have a fuel burn, but of course,
that's been obviously at sea level, and it's going to be very much different at
altitude. But from the Atlantic and the Pacific crossings, they've got a good
idea of what their fuel burns are likely to be at altitude. But it's the hot
air part of it, if you like, on the Pacific and Atlantic, being hot air
balloons, was significantly more than we're ever going to burn on the Global
helium balloon.
NOVA: With a third person in there, would that change some of the
variables as well?
EVERSFIELD: Not really, because we have a bigger balloon—I mean, the
helium is providing the lift. With the hot air balloon, the hot air provides
the lift and that hot air is always having to be fed into that balloon
continuously unless the balloon has been designed such that the solar radiation
during the day can heat the balloon sufficiently so you don't need to burn.
That is, in fact, what is done on quite a few balloons. But here with a helium
balloon, it will just maintain a reasonably constant altitude. There's no
energy input, as such. The balloon will just float. It's a lot easier to fly
and, in fact, a lot less input is required by the pilot.
NOVA: Do you have any fun experiments that kids or interested students
could try at home to convey some of the concepts we've discussed, using this
balloon adventure as a model?
|
Hear Eversfield
via RealAudio: 14.4 |
28.8 |
ISDN
|
EVERSFIELD: There is a nice little test that they can do, which is to
get a cork with a little bit of weight hanging on the cork and put it inside a
bottle with—like a champagne bottle, or if you're not as rich as we are, a
wine bottle—fill the bottle with water, put your little cork with the weight
on it inside the bottle, and it will probably just float. And then, when you
press the cork to close the bottle, as the pressure gets higher from the
compression of the air on the top of the bottle, so the cork with a little
weight will gradually sink and you can actually stop it, depending on how hard
you press on the cork on the top.
And that will explain what buoyancy is all about. Because really, we're
talking about buoyancy. It's like a submarine in the water. It's exactly the
same principle but we're doing it in air. So if you can look at how a
submarine works by filling a tank with water and it will sink and reach a
certain point ,then you can let some of that water out and it will rise, this
experiment shows that sort of principle of buoyancy, which is certainly how the
balloon works. It's simply a buoyancy experiment.
NOVA: Great, thank you.
EVERSFIELD: Thank you.
Interviews: Ackroyd | Branson | Erickson
| Eversfield | Kendrick
Photos: Aaron Strong
Global Contenders '97/'98 |
Expedition '96/'97 |
Fossett |
Virtual Flight
Science of Ballooning |
Teacher's Guide |
Resources |
Transcript |
Balloon Home
Editor's Picks |
Previous Sites |
Join Us/E-mail |
TV/Web Schedule
About NOVA |
Teachers |
Site Map |
Shop |
Jobs |
Search |
To print
PBS Online |
NOVA Online |
WGBH
© | Updated October 2000
|
|
|